- 1Key Lab of Biopesticide and Chemical Biology, Ministry of Education; State Key Laboratory of Ecological Pest Control for Fujian and Taiwan Crops, College of Life Sciences, Fujian Agriculture and Forestry University, Fuzhou, Fujian, China
- 2Department of Microbiology and Cell Science, Institute of Food and Agricultural Sciences, University of Florida, Gainesville, FL, United States
- 3Dulwich International High School Suzhou, Suzhou, Jiangsu, China
- 4Fujian Academy of Forestry, Fuzhou, Fujian, China
- 5College of Horticulture, Fujian Agriculture and Forestry University, Fuzhou, Fujian, China
Introduction: Tea is one of the most widely consumed beverages around the world. Larvae of the moth, Ectropis obliqua Prout (Geometridae, Lepidoptera), are one of the most destructive insect pests of tea in China. E. obliqua is a polyphagus insect that is of increasing concern due to the development of populations resistant to certain chemical insecticides. Microbial biological control agents offer an environmentally friendly and effective means for insect control that can be compatible with “green” and organic farming practices.
Methods: To identify novel E. obliqua biological control agents, soil and inset cadaver samples were collected from tea growing regions in the Fujian province, China. Isolates were analyzed morphologically and via molecular characterization to identity them at the species level. Laboratory and greenhouse insect bioassays were used to determine the effectiveness of the isolates for E. obliqua control.
Results: Eleven isolates corresponding to ten different species of Metarhizium were identified according to morphological and molecular analyses from soil and/or insect cadavers found on tea plants and/or in the surrounding soil sampled from eight different regions within the Fujian province, China. Four species of Metarhizium including M. clavatum, M. indigoticum, M. pemphigi, and M. phasmatodeae were documented for the first time in China, and the other species were identified as M. anisopliae, M. brunneum, M. lepidiotae, M. majus, M. pinghaense, and M. robertsii. Insect bioassays of the eleven isolates of Metarhizium revealed significant variation in the efficacy of each isolate to infect and kill E. obliqua. Metarhizium pingshaense (MaFZ-13) showed the highest virulence reaching a host target mortality rate of 93% in laboratory bioassays. The median lethal concentration (LC50) and median lethal time (LT50) values of M. pingshaense MaFZ-13 were 9.6 × 104 conidia/mL and 4.8 days, respectively. Greenhouse experiments and a time-dose-mortality (TDM) models were used to further evaluate and confirm the fungal pathogenic potential of M. pingshaense MaFZ-13 against E. obliqua larvae.
Discussion: Isolation of indigenous microbial biological control agents targeting specific pests is an effective approach for collecting resources that can be exploited for pest control with lowered obstacles to approval and commercialization. Our data show the presence of four different previously unreported Metarhizium species in China. Bioassays of the eleven different Metarhizium strains isolated revealed that each could infect and kill E. obliqua to different degrees with the newly isolated M. pingshaense MaFZ-13 strain representing a particularly highly virulent isolate potentially applicable for the control of E. obliqua larvae.
1. Introduction
Ectropis obliqua Prout is a Lepidopteran moth species that is one of the most common insect pests found on the leaves of tea trees in China (Zeng et al., 2018). Infestations can reduce crop quality and yields, especially along the middle and lower reaches of the Yangtze River (Yan et al., 2020). Ectropis obliqua is a polyphagous insect with a variety of different hosts. In addition to tea, it can also cause damage to soybean [Glycine max (Linn.) Merr.], sesame (Sesamum indicum L.), and even cultivated flowers (e.g., Chrysanthemum morifolium Ramat. and the common sunflower Helianthus annuus L.) (Yang et al., 2011; Li et al., 2019).
With respect to the often hilly tea gardens, cultivation of a single tea variety coupled with optimal humidity and light (sun) cycles often leads to high infestation levels and significant damage to leaves that are still tender, moist and closed, and hence a perfect habitat for the insect, and one that makes control more difficult. The first generation of E. obliqua usually takes place in early April, followed by population recurrences every 6–8 weeks (Zhang et al., 2022).
Chemical, biological, and physical control methods have all been applied for suppressing E. obliqua populations (Idris et al., 2020). However, overuse of chemical pesticides has led to the emergence of resistance, resurgence, and residue (3R) issues (Zeng et al., 2018). This, coupled with increasing demand for organic tea, has resulted in a pressing need for the identification and development of efficient, low residue, low (non-target) toxicity control approaches for E. obliqua that optimally should be compatible with “green” or organic farming practices (Soh and Veera Singham, 2021). One such approach is the identification of biological control agents that has the advantages of being (i) more environmentally friendly, (ii) less harmful to humans and other animals including beneficial insects (e.g., bees and spiders), and (iii) possess decreased chances for the development of resistance (to the control method), as compared to chemical insecticides. Hence, the application of biological control is increasing for many different crop systems, especially as part of integrated pest management (IPM) practices. Nuclear polyhedrosis virus (EoNPV) and Bacillus thuringiensis (Bt) are currently the two most widely used biological control agents targeting E. obliqua (Ma et al., 2007; Idris et al., 2020). In addition, some plant-based insect toxins have been used to control E. obliqua, including a tea saponin that has been shown to be able to kill E. obliqua larvae without causing harm to non-target organisms such as Ebrechtella tricuspidata Fahricius and Evarcha albaria L. Koch (Zeng et al., 2018; Zhao et al., 2020). However, many of these approaches are costly, and application can be difficult and time-consuming. Entomopathogenic fungi represent an alternative method for targeting insects that can be cost-effective, capable of controlling target populations in diverse habitats, are naturally occurring, and have shown little, if any, development of resistance to the fungus by insect targets as a result of application (Glare et al., 2012; Ortiz-Urquiza et al., 2015; Gao et al., 2017).
Entomopathogenic fungi invade healthy insects via penetration of the exoskeleton, proliferation within the host body, tissue dissolution, and ultimately host death (Ortiz-Urquiza and Keyhani, 2013; Han et al., 2020; Mahato and Paikaray, 2021). There are over 100 genera and more than 1,000 species of entomopathogenic fungi described thus far (Mongkolsamrit et al., 2020; Chen et al., 2021). Metarhizium is one of the most important groups and includes more than 80 species (e.g., Metarhizium anisopliae (Metschn.) Sorokin, Metarhizium acridum (Driver and Milner) J.F. Bisch., S.A. Rehner and Humber, Metarhizium robertsii J.F. Bisch., S.A. Rehner and Humber, Metarhizium clavatum Luangsa-ard, Mongkols., Lamlertthon, Thanakitp. and Samson, Metarhizium indigoticum (Kobayasi and Shimizu) Kepler, S.A. Rehner and Humber, and Metarhizium pemphigi (Driver and Milner) Kepler, Humber and S.A. Rehner). Some of these are species that are cosmopolitan and have a broad host range as well as those that have narrower host ranges. For example, M. anisopliae can infect > 200 different species of insects throughout the world, while M. acridum is mainly a pathogen of members of the Acrididae. The former is used in a wide variety of crop applications, whereas the latter has been commercialized mainly for the control of locusts (Mongkolsamrit et al., 2020; St Leger and Wang, 2020; Kamga et al., 2022).
Known entomopathogenic fungi that can target E. obliqua include Paecilomyces spp., Fusarium spp., and Erynia spp. as well as Beauveria bassiana (Balsamo) Vuillemin and Metarhizium anisopliae (Idris et al., 2020; Wang et al., 2020; Zhang et al., 2020). However, aside from M. anisopliae, other species of Metarhizium infecting E. obliqua have yet to be reported. Here, we report on the field isolation and screening of entomopathogenic fungi, identifying 11 Metarhizium isolates belonging to 10 different species. Our data provide records for species resources, identify new taxa in China, and examine the biological control potential of all of the isolates against E. obliqua larvae as compared with the reference M. anisopliae (CQMa421) strain currently commercialized for a range of target insects. These data identify one isolate, M. pingshaense MaFZ-13, as particularly effective in laboratory bioassays toward E. obliqua, and extend these results to greenhouse experiments that confirm the effectiveness of the fungal strain for E. obliqua control.
2. Materials and methods
2.1. Fungal acquisition
Eleven fungal isolates were gathered from moribund insect cadavers or obtained from soil samples in Fujian Province (23°33′-28°19′N, 116°15′-120°43′E), China. Collection sites encompassed a range of tea-growing areas including the Wuyi Mountain Nature Reserve of Wuyi city, Wanmulin Nature Reserve of Jian'ou city, Taimu Mountain Nature Reserve of Ningde city, Huangchu Forest Nature Reserve, Gushan Mountain and Fuzhou National Forest Park of Fuzhou city, Niumulin Nature Reserve of Quanzhou city, and Hubo Liao Nature Reserve of Zhangzhou city (Supplementary Figure S1).
2.2. Fungal isolation
Field-collected insect cadavers, typically fully covered with fungal mycelia, and soil samples were collected from the different natural reserves. Collected insects were cut or clipped with a knife or tweezers on a sterile worktable (Airtech SW-CJ-2FD). Samples were soaked in 75% alcohol for 30–60 s, rinsed in sterile water for 10–15 s, then placed on a clean glass slide, and slightly smashed with a clean blade or the back of the blade handle until the base was crushed, after which 200 μL of sterile distilled dH2O was added, mixed with the crushed materials, and then placed onto potato dextrose agar (PDA, Difco TM) Petri dishes (Bioshap) and cultured at 25 ± 1°C and 60% relative humidity (RH) for 10–14 days in an incubator (Binder BD240). Soil samples were serially diluted using sterile ddH2O and spread onto PDA plates. Colonies were assessed after 3–5 d growth, and single colonies were gently picked and streaked onto fresh culture media for several rounds until the resultant single colonies were homogeneous in appearance (Kumar et al., 2018; Wei et al., 2019).
2.3. Identification of fungi
2.3.1. Morphological identification
Single colony purified strains were inoculated in the center of PDA plates and allowed to grow. Asexual characteristics, including the production and morphology of phialides, mycelia, and conidia, were examined by inoculating spores on glass slides containing a thin layer of PDA (5–10 mm in diameter) and overlaid with a cover slip (Kepler et al., 2014; Mongkolsamrit et al., 2020). Slides were placed in Petri dishes with a small amount of water to maintain humidity and kept at room temperature to visualize the development of phialides and conidia. Where indicated, 1–2 drops of cotton blue were added to the slide, and sample blocks were encapsulated with colorless transparent nail polish for further observation. Micromorphological images of the fungal cells (e.g., size and shape) were collected using a Nikon Eclipse 80i microscope (Nikon) attached to a digital camera. Twenty to thirty individual length and width measurements were taken for each sample, with absolute minima and maxima. Mycelia were inoculated on PDA plates and incubated at 25°C with colonies photographed and growth diameters measured as indicated (Kepler et al., 2014; Mongkolsamrit et al., 2020).
2.3.2. Gene amplification and molecular phylogenetic analysis
The Omega Bio-Tek fungal kit was used to extract fungal total DNA, and primers were used to amplify (1) transcription elongation factor (TEF) and (2) the RNA polymerase II largest subunit 1 (RPB1) gene fragments of the fungal strains based on previous reports (Kepler et al., 2014; Senthil Kumar et al., 2021). Primers 983F (5′-GCYCCYGGHCAYCGTGAY TTYAT-3′) and 2218R (5′-ATGACACCRACRGCRACRGTYTG-3′) were used for the TEF gene, and primers RPB1 (cRPB1Af 5′-CAYCCWGGYTTYATCAAGAA-3′ and RPB1Cr 5′-CCNGCDATNTCRTTRTCCATRTA-3′) were used for RBP1 amplification (Senthil Kumar et al., 2021). In brief, polymerase chain reaction (PCR) experiments were performed as follows: (1) for TEF amplification: 95°C for 10 min, followed by 40 cycles at 94°C for 30 s, 55°C for 30 s, 72°C for 1 min, and a final extension step at 72°C for 10 min; (2) for RPB1 amplification: 95°C for 5 min, followed by 40 cycles at 95°C for 1 min, 50°C for 2 min, 72°C for 2 min, and a final extension step at 72°C for 10 min. The resultant PCR products were sequenced at Boshan Company, China. The generated sequences were submitted to NCBI GenBank and used for multi-gene phylogenetic analysis. The sequences were employed as the query to perform a BLAST search (http://blast.ncbi.nlm.nih.gov/), and the sequence similarity studies were performed using ClustalX. Phylogenetic trees were constructed with the method of Bayesian inference (BI) using MrBayes software (Portela et al., 2021).
2.4. Insect breeding
A laboratory colony of E. obliqua was established using late instar larvae gathered from an infested tea plantation in Fuzhou National Forest Park, Fuzhou, China. Insects were fed on the fresh tender tea leaves and maintained in plastic rearing cages (30 × 30 × 30 cm) and kept at 25 ± 2°C, 70–80% relative humidity (RH) with 10:14 h (day: night) photoperiod until pupation. Pupae were gently picked from leaves and transferred into a spawning container (15 × 15 × 20 cm) and raised with 10% honey water until eclosed adults were seen. After adult emergence and mating, the adults were allowed to lay eggs on fresh young tea leaves grown inside the cages. Larvae were reared on the new tender leaves after hatching and collected for use in bioassays at the second instar stage (Lu et al., 2020).
2.5. Virulence bioassays
2.5.1. Conidial preparation
All fungal strains were inoculated on PDA medium and incubated in an incubator at 25 ± 1°C and 60% RH for 14 days. Spores produced on PDA Petri dishes were harvested into sterile distilled water containing 0.05% Tween 80 (Sigma-Aldrich), and the conidial solution was mixed until uniform. The spore suspension was then filtered through 4–8 layers of lens paper and spore concentrations were determined using a hemocytometer. Spore concentrations were then adjusted as indicated. For the determination of the fungal median lethal concentration to kill 50% of insect hosts, (LC50), five concentrations of fungal spores: 1 × 104, 1 × 105, 1 × 106, 1 × 107, and 1 × 108 conidia/mL, were used. For calculation of the median lethal time to kill 50% of the insect hosts, (LT50), a suspension of 1 × 108 conidia/mL was used.
2.5.2. Insect bioassays
Ectropis obliqua 2nd instar larvae were placed on fresh tea leaves, and spore suspension adjusted to different concentrations as above was evenly sprayed onto the surface of the test insect, which was then stored and maintained in a sterilized feeding cage (AIPUINS) after allowing the insects to air dry after treatment. Each treatment consisted of 20 larvae × three technical replicates for each group. Sterile distilled water containing 0.05% Tween 80 only was served as a control. The feeding cages were continuously cultured in a greenhouse at 25 ± 2°C, relative humidity (70–80%), and 10:14h (day: night) for lab assay. The number of dead larvae (surface melanization of insect cadaver, followed by sporulation) was counted daily after 24 h of post-inoculation, and the test was terminated 13 days after treatment. The dead larvae were picked out and placed in a clean plastic petri dish without media to observe fungal sporulation. The mortality rate of the control group was <10% (Im et al., 2022). The entire experiment was repeated three times with different batches of insects and spores.
2.5.3. Greenhouse experiments
Three greenhouse trials with M. pingshaense were conducted from March to November 2022, respectively, in E. obliqua-infested tea seedlings planted in the greenhouse (temperature 25 ± 2°C, 70–80% RH, 10:14 h day: night). The 2nd instar larval numbers of E. obliqua per seedling were counted with a counter before fungal spray (Beloti et al., 2015). The fungal spore suspensions (1 × 104, 1 × 105, 1 × 106, 1 × 107, and 1 × 108 conidia/mL) were prepared in sterile distilled water containing 0.05% Tween 80 (Sigma-Aldrich). The spore suspensions of 500 mL at the above different concentrations were used to thoroughly spray each tea tree (n = 20) that was naturally infested with larvae of E. obliqua with the aid of 2-liter pump action handheld sprayer (Senthil Kumar et al., 2021). Twenty tea seedlings sprayed with sterilized water containing 0.05% Tween 80 acted as a control (Beloti et al., 2015). Mortality data were counted daily according to the treated and control plants and terminated after 10 days of treatment (Senthil Kumar et al., 2021). The greenhouse trials were repeated three times, and greenhouse bioassays were performed in triplicate at different time intervals. The mortality rates were calculated using the recorded data from each treatment as mentioned above.
2.6. Statistical analysis
Data were transformed by arc sine [(x + 0.5)/100] ½ before analysis (Senthil Kumar et al., 2021). Data on conidial concentration, time, and their interactive effects on insect mortality rate were analyzed using GLM. Mortality data of Ectropis oblique were corrected using Abbott's formula. Median lethal concentration (LC50) and median lethal time (LT50) values were calculated by DPS software based on the binomial GLM function. Biocontrol efficacy data were estimated with the aid of the Student's t-test and TDM model (Tang and Zhang, 2013; Lian et al., 2021).
3. Results
3.1. Species identification of Metarhizium spp.
3.1.1. Morphological identification
A total of 11 Metarhizium isolates, collected from fungal-infected cadavers of insects found on tea [Camellia sinensis (Linnaeus) Kuntze] leaves and from soil samples from various tea-growing regions in the Fujian Province of China, were single colony purified, and their morphology and the molecular sequences for the TEF and RBP1 genes were determined as detailed in the Methods section (Kepler et al., 2014; Mongkolsamrit et al., 2020). A summary of the host/origin, GenBank accession numbers for the cloned TEF and RBP1 regions, and species assignments for the 11 Metarhizium isolates are given in Supplementary Table S1. Isolates were plated onto PDA, and sporulation for all isolates was observed beginning ~4–6 days after initial inoculation. Morphological characteristics (Supplementary Table S2) were recorded as follows:
3.1.1.1. Metarhizium anisopliae
Fungal colonies grown on PDA at 25°C for 14 days, which had a diameter of 50 mm, initially seemed white and gradually turned greenish olive, with the reverse side dark yellow to white. Sporulation for all isolates was observed beginning ~4–6 days after initial inoculation. Conidiophores were born in aerial mycelia and were smooth, terete to clave-like, apically branched, 2–3 phialides on per branch, (5–) 7.0–9.0 (−10.5) × 2–2.5 (−3) μm (Kepler et al., 2014). Conidia were cylindrical or clavate, smooth-walled, adhering in globose to cylindrical head at the apex of phialides, (5–) 6.0–8.0 (−9) × 2–2.5 (−3) μm (Figures 1A–C).
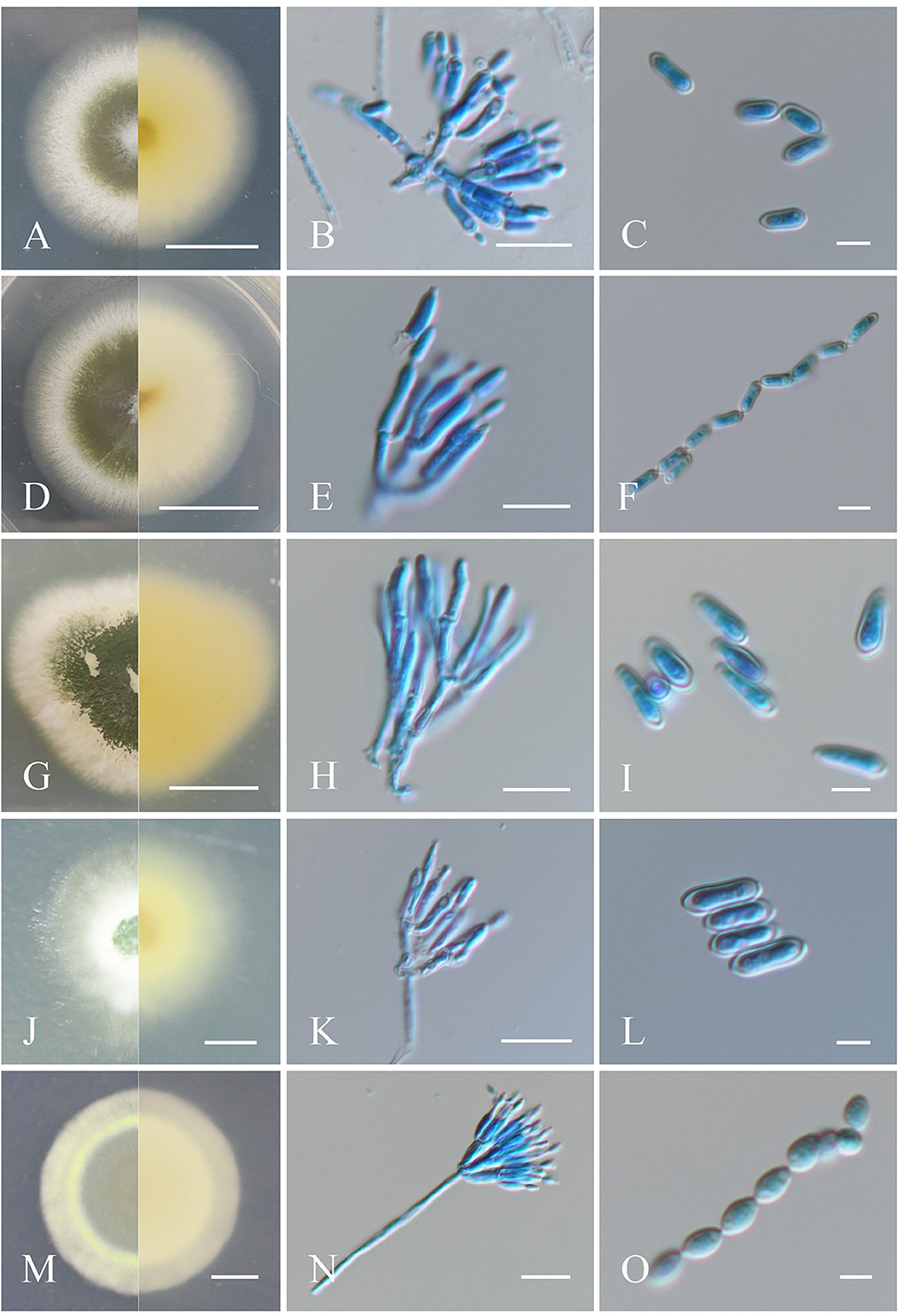
Figure 1. Morphological characteristics of the isolates of Metarhizium. (A, D, G, J, M) Colonies of M. anisopliae, M. pingshaense, M. robertsii, M. majus, and M. brunneum cultured on PDA at 25°C for 14 days; (B, E, H, K, N) spore production structures of each isolate stained with cotton blue, respectively; (C, F, I, L, O) conidial morphology of each isolate stained with cotton blue, respectively. Scale bars: (A, D, G, J, M) = 0.5 cm; (B, E, H, K, N) = 10 μm; (C, F, I, L, O) = 5 μm.
3.1.1.2. Metarhizium pingshaense
Colonies of the fungus cultured on PDA, which had a diameter of 48 mm at 25°C for 14 days, initially appeared white and gradually changed dark green to olive after 4–6 days of sporulation, with the reverse light yellow to white (Mongkolsamrit et al., 2020). Conidial production was concentrated at the center of the colony and formed a concentric circular pattern toward the end. Conidiophores were born in aerial mycelia and were smooth, terete, apically branched, 1–3 phialides on per bifurcation, (5–) 6.5–8.5 (−10) × 2–3 μm. Conidia were cylindrical or clavate in shape, formed in long chains, and appeared smooth-walled, (5–) 6.0–7.0 (−8.5) × 1.5–2 (−2.5) μm (Figures 1D–F).
3.1.1.3. Metarhizium robertsii
Fungal colonies formed on PDA, with a diameter of 31 mm at 25°C for 14 days, changed from flocky and white cream to green over time, with pale yellow on the reverse side after sporulation (Mongkolsamrit et al., 2020). Conidiophores emerged on aerial hyphae and were branched, with smooth walls. Phialides were cylindrical and slightly curved at the top, (5–) 6.5–8.5 (−11) × 1.5–2 μm. Conidia were clavate, smooth-walled, adhering in globose to cylindrical heads at the apex of phialides, (5.0–) 7.0–8.0 (−9.0) × 2–2.5 (−3) μm (Figures 1G–I).
3.1.1.4. Metarhizium majus
Fungal colonies incubated on PDA at 25°C for 14 days were ~29 mm in diameter and were initially white and hairy, progressively developing into dark green due to conidia production, with light yellow to white on the reverse (Mongkolsamrit et al., 2020). Conidiophores were apically branched, with 2–3 phialides. Phialides were cylindrical in shape with semi-mastoid tips, (6.0–) 7–9 (−10) × 2–3 μm. Conidia were cylindrical and smooth-walled, (5–) 6.5–8.0 (−10) × 2.5–3 μm (Figures 1J–L).
3.1.1.5. Metarhizium brunneum
Colonies on PDA at 25°C for 14 days were ~30 mm in diameter, originally appearing white and turning grayish-green upon sporulation, with white to pale yellow coloration on the reverse. Conidiophores were generated on aerial mycelium and were smooth, apically branched, and cylindrical, each with 2–3 phialides. Phialides walls were smooth, cylindrical to fusiform in shape, and slightly raised at the top, (4.5–) 5–6 (−7) × 1.5–2 μm. Conidia were smooth-walled, oval in shape, and formed in long chains, 3.5–4 (−5) × 2–2.5 (−3) μm (Mongkolsamrit et al., 2020; Figures 1M–O).
3.1.1.6. Metarhizium clavatum
Fungal colonies on PDA medium at 25°C for 14 days were ~17 mm in diameter and gradually changed from white to yellowish green upon sporulation, with the reverse side orange-yellow. Conidiophores were generated on branches and formed palisade layers. Phialides were cylindrical with a semi-mastoid tip, (4–) 6–8 (−9) × (1.5–) 2–3 μm (Mongkolsamrit et al., 2020). Conidia were cylindrical, smooth-walled, and formed in long chains, adhering in globose to cylindrical heads at the apex of phialides, (4–) 5–6 (−8) × 2.5–3 μm (Figures 2A–C).
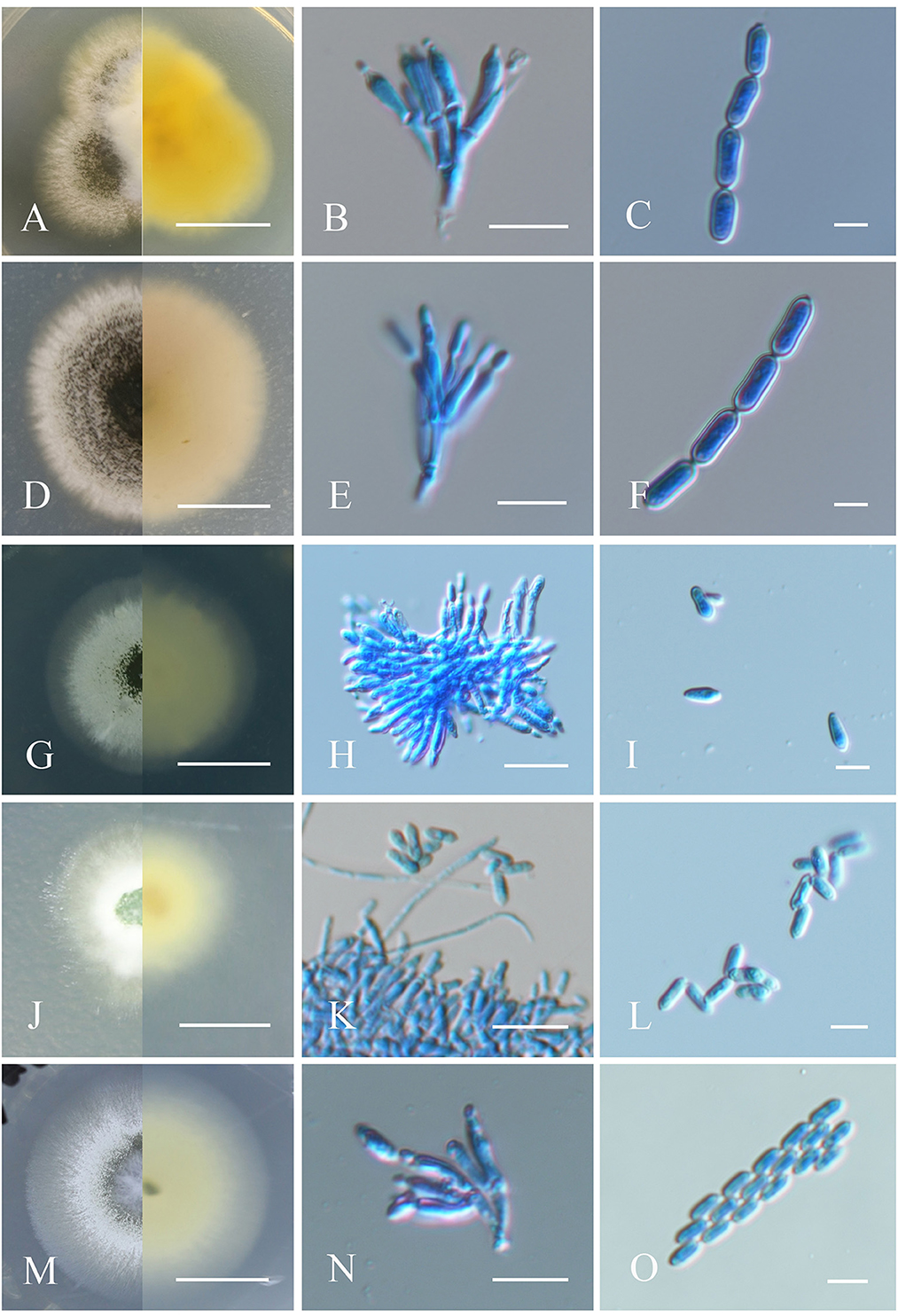
Figure 2. Morphological characteristics of the isolates of Metarhizium. (A, D, G, J, M) Colonies of M. clavatum, M. indigoticum, M. phasmatodeae, M. pemphigi, and M. lepidiotae cultured on PDA at 25°C for 14 days; (B, E, H, K, N) spore production structures of each isolate stained with cotton blue, respectively; (C, F, I, L, O) conidial morphology of each isolate stained with cotton blue, respectively. Scale bars: (A, D, G, J, M) = 0.5 cm; (B, E, H, K, N) = 10 μm; (C, F, I, L, O) = 5 μm.
3.1.1.7. Metarhizium indigoticum
Fungus colonies on PDA at 25°C originally appeared white, villous, and aerial and gradually became dark brown and velvety, with radial cracks and nearly colorless reverse after 4–6 days of sporulation, with a colony diameter of ~30 mm after 14 days. Conidiophores are apically branched, each with 2–3 phialides (Kepler et al., 2014). Phialides were cylindrical or flask-shaped with a half mastoid tip, 6–8 (−9) × 2–2.5 (−3) μm. Conidia were oval, smooth-walled, and formed in long chains, 6.5–8 (−9.5) × 2–3 μm (Figures 2D–F).
3.1.1.8. Metarhizium phasmatodeae
Colonies cultivated on PDA medium initially appeared white, smooth, and cottony and progressively turned leaf green to olive upon sporulation with pale yellow reverse coloring, and a colony diameter of ~40 mm after 14 days. Conidiophores were produced on aerial mycelium and were smooth, cylindrical, and branched (Mongkolsamrit et al., 2020). Phialides were smooth-walled, cylindrical to clubbed in shape, and slightly opacified at the top, (5–) 7.0–9.0 (−10) × 2–3 μm. Conidia were cylindrical or clavate, smooth-walled, adhering in globose to cylindrical head at the apex of phialides, (5–) 6.5–8.0 (−9) × 2–2.5(−3) μm (Figures 2G–I).
3.1.1.9. Metarhizium pemphigi
Colonies on PDA originally appeared white and cottony and gradually turned light green and leaf green with pale yellow reverse side after producing spores, and a colony diameter of ~20 mm after 14 days (Kepler et al., 2014). Conidiophores formed on aerial mycelium and were smooth and cylindrical in shape, 5–6 (−7) × 1.5–2 μm. Conidia were cylindrical or clavate, smooth-walled, adhering in globose to cylindrical heads at the apex of phialides, 4–5 (−6) × 1.5–2 μm (Figures 2J–L).
3.1.1.10. Metarhizium lepidiotae
Colonies on PDA were initially white, flocculent, and cotton-like and gradually turned dark brown after sporulation with pale yellow coloring on the reverse, and a colony diameter of ~25 mm after 14 days. Conidial generation was denser in the middle of the colony and formed a concentric circular pattern toward the end. Conidiophores occurred on aerial hyphae and were smooth-walled, with apical branches, and 2–3 phialides per branch. Phialides were smooth-walled, clave-like with semi-mastoid tips, (6–) 6.5–9.5 (−10) × 2–3 μm. Conidia were smooth-walled, cylindrical, and grew in long parallel chains, 5–6 (−7) × 2–2.5 μm (Mongkolsamrit et al., 2020; Figures 2M–O).
3.1.2. Molecular phylogeny
The partial nucleotide sequences of the TEF and RBP1 conserved regions of the fungal isolates were amplified in the present study to validate their morphological identities and deposited at GenBank with accession numbers (Supplementary Table S1). BLAST analyses of generated sequences exhibited high sequence similarity with different species of Metarhizium. A phylogenetic tree of Metarhizium at the species level was constructed based on the Bayesian inference (BI) method using a combined data set of RPB1 and TEF, with a combined gene length of 1,102 bp. Metarhizium anisopliae MaYTTR-04-04, MaXJ-04-1 and MaFZ-13 of M. pinghaense, M. brunneum MaTGZ-01, M. robertsii MaQZ-02, M. clavatum MaNATR-02, M. indigoticum MaTK-01, M. majus MaXJ-06, M. lepidiotae MaQL-02, M. pemphigi MaFZ-11, and M. phasmatodeae MaJO-01 were grouped in their individual species clades, with the 11 test isolates clearly identified within the 10 established species (Figure 3). Notably, M. clavatum, M. indigoticum, M. pemphigi, and M. phasmatodeae were newly discovered and recorded in China for the first time. Overall, the 11 Metarhizium isolates were placed into 10 different species, with two exemplars of M. pingshaense.
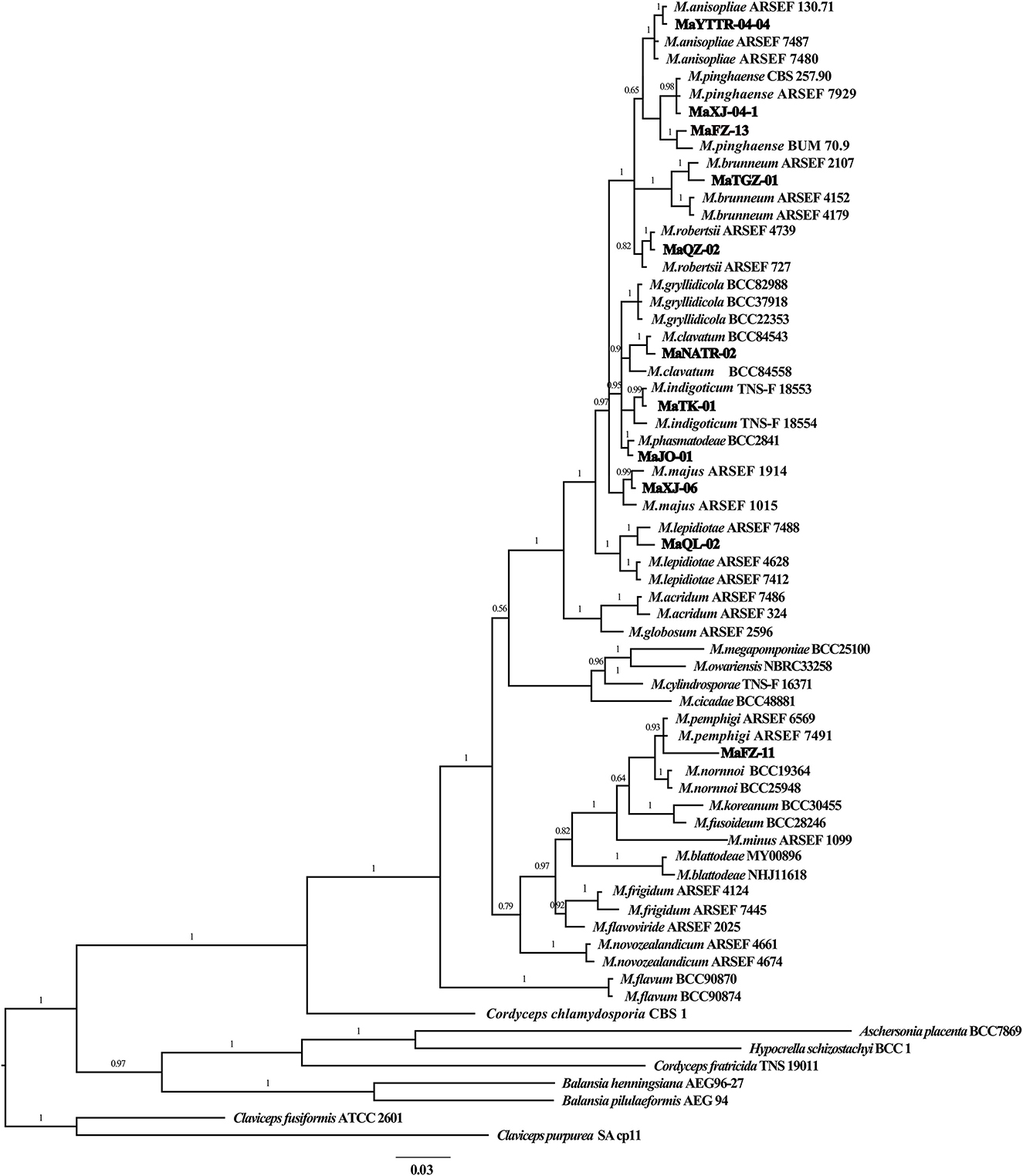
Figure 3. Phylogenetic tree of Metarhizium constructed using Bayesian inference analyses of combined TEF and RPB1 sequence data.
3.2. Bioassay
Preliminary fungal screening using E. obliqua 2nd instar larvae and fungal spore concentrations of 5 × 109 conidia/mL led to 100% pest mortality 10 days post-inoculation for three randomly selected Metarhizium isolates tested. Infected insects initially showed melanization (1–2 d post-inoculation, Figure 4A), followed by a gradual covering of the body surface with white hyphae (2–4 d post-inoculation, Figure 4B), ultimately resulting in the whole body of the insect cloaked with thick layers of green conidia (3–5 days after insect death, Figure 4C).
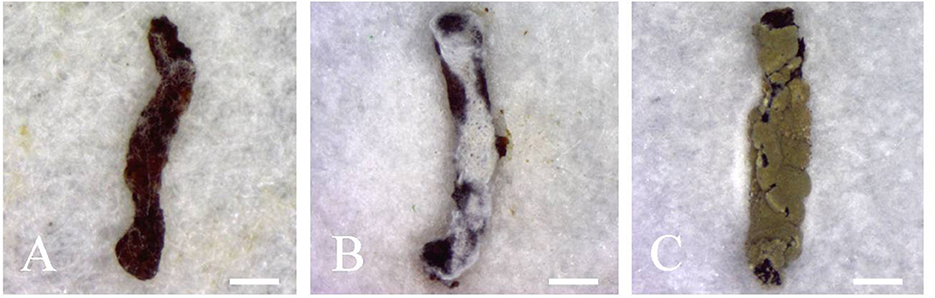
Figure 4. Infected second instar larvae of E. obliqua showing (A) melanization (1–2 d post-inoculation), (B) proliferation of hyphae on the surface of the insect (2–4 d post-inoculation), and (C) massive sporulation on the insect corpses. Scale bars: (A–C) = 1 mm.
A second round of bioassays using E. obliqua 2nd instar larvae and fungal spore concentrations of 1 × 108 conidia/mL was carried out over the course of 13 days (Figure 5). Among these, infection using M. pingshaense MaFZ-13 resulted in the highest mortality toward E. obliqua larvae reaching 93%, followed by that of M. anisopliae MaYTTR-04-04 (83% mortality) and M. pingshaense strain MaXJ-04-1 (79% mortality). Metarhizium clavatum MaNATR-02 showed the lowest virulence to E. obliqua with approximately 40% mortality after 13 days post-inoculation, with several others showing similar levels of mortality ranging from 40 to 60% of the target insects killed within the bioassay time frame, and those of the other species of Metarhizium fell between the two. The calculation of LT50 values confirmed the highest virulence for M. pingshaense MaFZ-13, LT50 = 4.8 ± 0.1 d, and lowest for M. indigoticum MaTK-01, LT50 = 9.9 ± 0.3 d (Table 1). Additional bioassays were performed in order to calculate the mean lethal concentration to kill 50% (LC50) of the target insect 10 days post-inoculation using different conidial concentrations ranging from 1 × 104 to 1 × 108 conidia/mL (Figure 6; Table 2). The LC50 for M. pingshaense MaFZ-13 was calculated to be LC50 = 9.6 ± 0.4 × 104 conidia/mL, with LC50 values for other strains = 1.3 ± 0.2 × 108 conidia/mL (M. brunneum MaTGZ-01, lowest virulent strain), and ranging from 5.2 ± 0.2 × 105 to 4.8 ± 0.3 × 107 conidia/mL for the remaining strains. For comparative purposes, the commercially available M. anisopliae strain CQMa421 was used in bioassays against E. obliqua 2nd instar larvae, showing an LT50 = 5.2 ± 0.2 d and an LC50 = 8.5 ± 0.6 × 105 conidia/mL (Tables 1, 2). The highest mortality was seen for the isolate corresponding to M. pingshaense, suggesting that it may hold promise for E. obliqua control with greater efficiency than current strains.
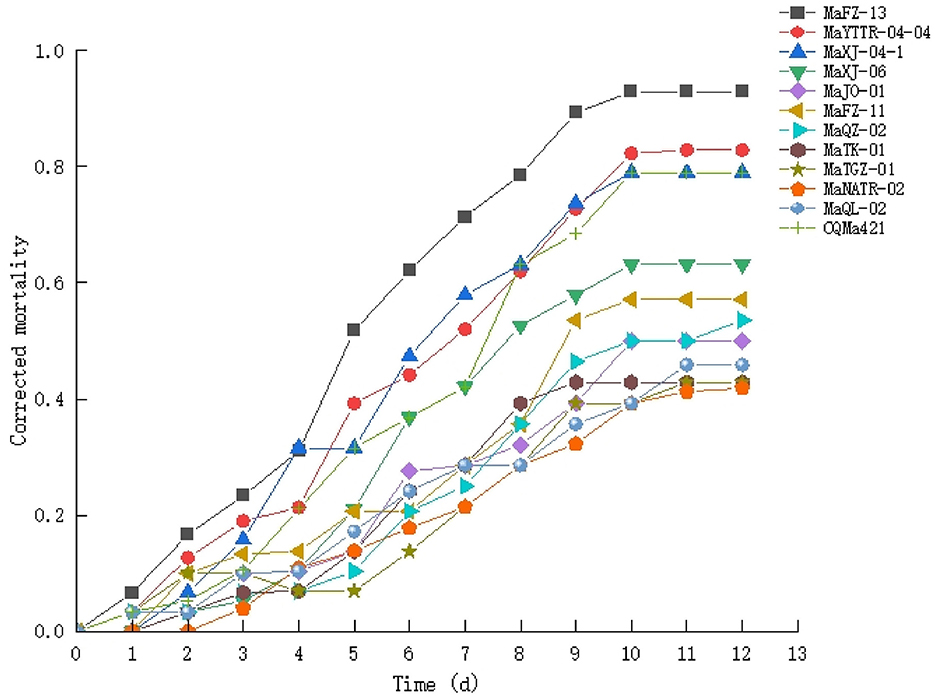
Figure 5. Time course of insect bioassays using the isolates of Metarhizium (1 × 108 conidia/ml) and E. obliqua 2nd instar larvae as the host. Experiments were performed in triplicate. Error bars = ±SE.
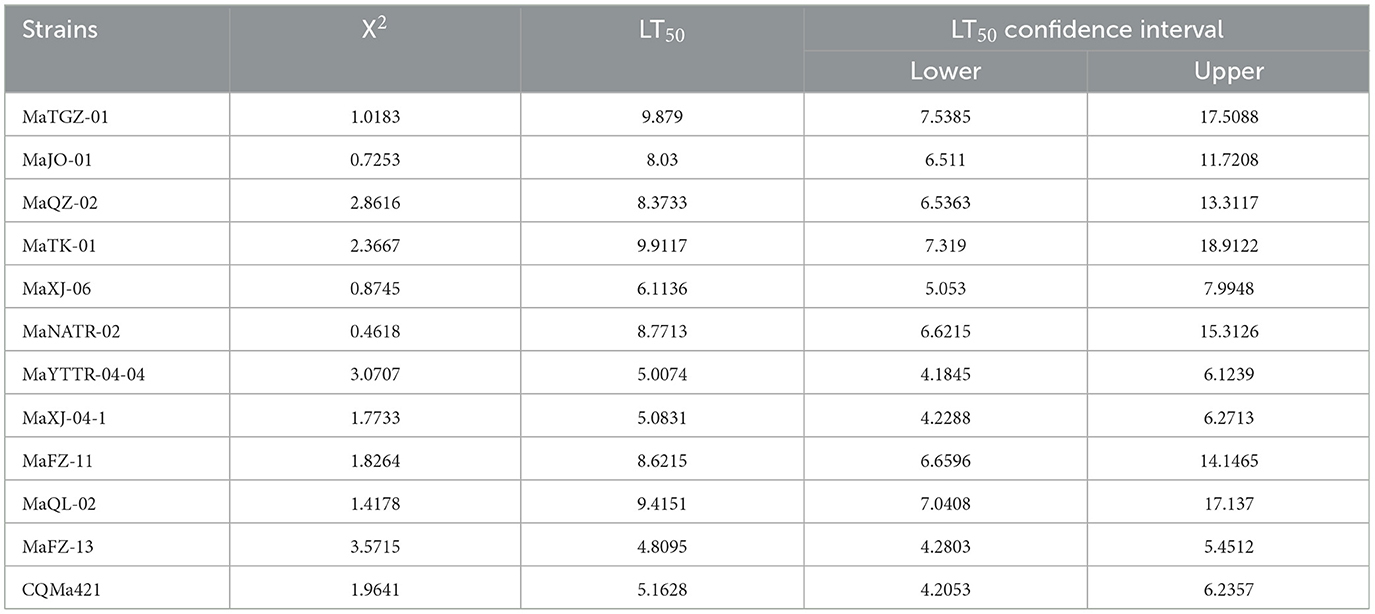
Table 1. Calculated mean lethal time (LT50) values of different strains of Metarhizium against 2nd instar E. obliqua larvae using 1 × 108 conidia/mL.
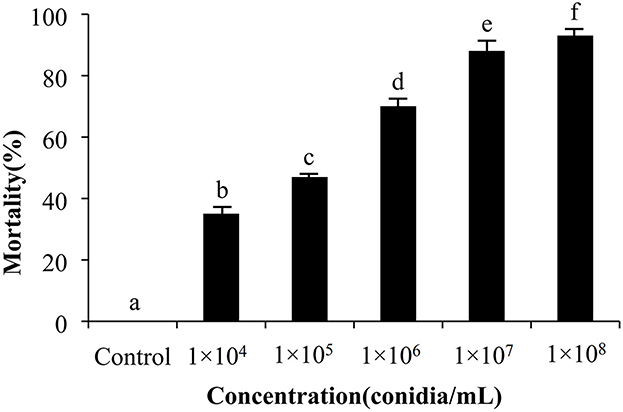
Figure 6. Concentration course of insect bioassays using the M. pingshaense (1 × 104 to 1 × 108 conidia/ml) and E. obliqua 2nd instar larvae as the host. Experiments were performed in triplicate. Error bars = ± SE. Mortalities followed by different letters of alphabets are significantly different at P < 0.05.
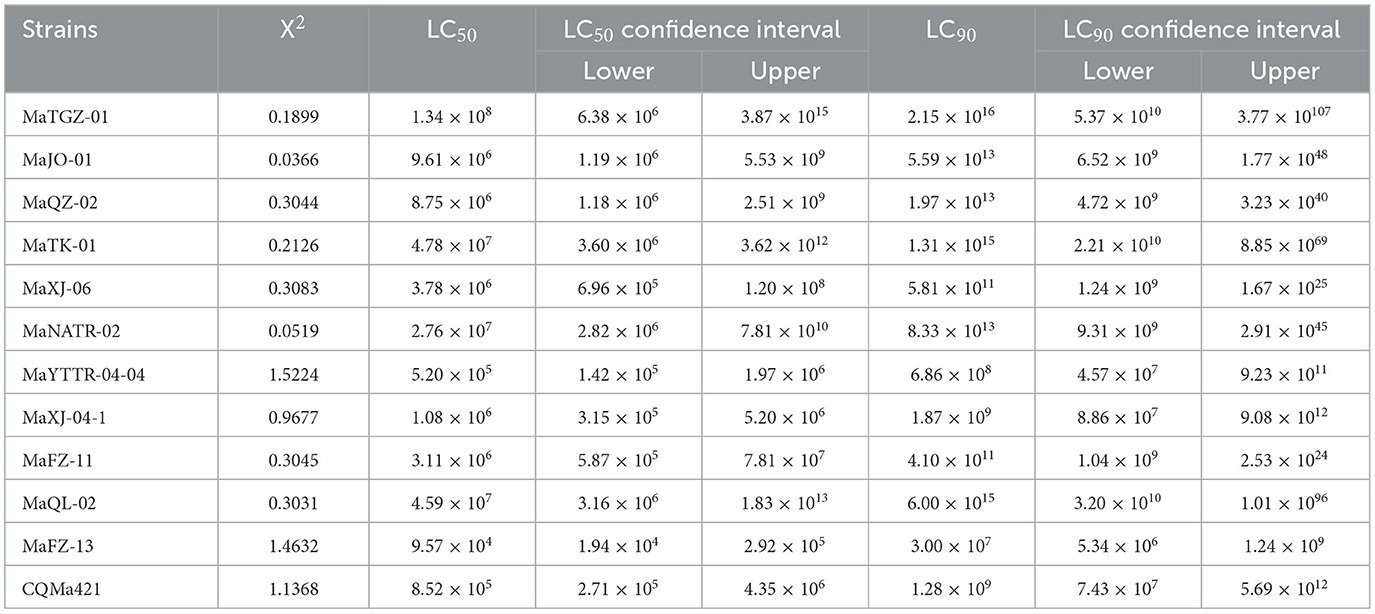
Table 2. Calculated mean lethal concentration (LC50 and LC90) values of different strains of Metarhizium against 2nd instar E. obliqua larvae.
3.3. Greenhouse trial and TDM evaluation of MaFZ-13
The cumulative mortality of E. obliqua larvae was recorded over a 10-day period of treatment with different concentrations (1 × 104 to 1 × 108 conidia/mL) of M. pingshaense MaFZ-13 in a greenhouse setting as detailed in the Methods section. The population of E. obliqua larvae decreased by 93, 78, 65 43%, and 28% 10 days post-inoculation using 1 × 108, 1 × 107, 1 × 106, 1 × 105, and 1 × 104 conidia/mL, respectively.
A time–dose–mortality (TDM) model was used to simulate and analyze the obtained data, with a data processing system (DPS) used to analyze the time–dose–mortality data of MaFZ-13 (Table 3). These data show that M. pingshaense MaFZ-13 (chi = 0.9943, df = 8, p = 0.99828, P > 0.05) conforms to Hosmer–Lemeshow fit heterogeneity test, indicating that there is no significant difference between the predicted value and the practical value and accords with TDM model. Overall, the edge lines in the three-dimensional model directly reflected the maximum death possibility of the tested fungus (Figure 7), confirming the high virulence of M. pingshaense MaFZ-13 toward E. obliqua.
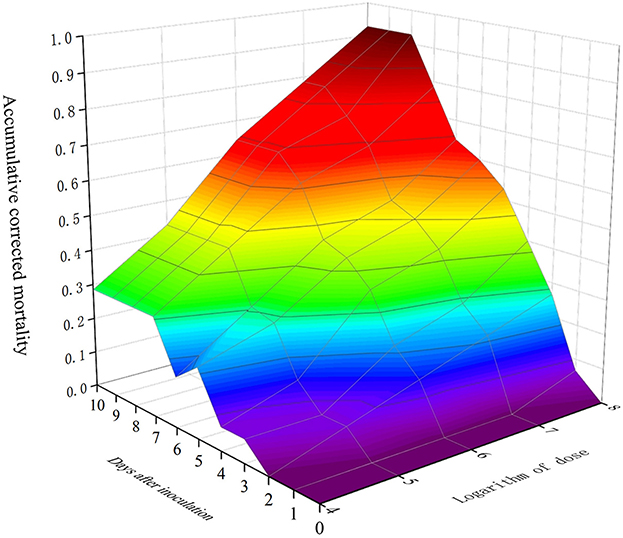
Figure 7. Three-dimensional plot mortality, time course, and fungal dose applied to a TDM model of M. pingshaense MaFZ-13 against E. obliqua in greenhouse experiments.
4. Discussion
The brewing of tea, Camellia sinensis, is one of the oldest and most popular beverages consumed worldwide. However, a large variety of different insects and pests can infest tea crops resulting in both direct damage and indirect damage via transmission of a range of microbial tea pathogens, which can lead to substantial economic losses (Chen and Chen, 1989). Significant efforts have been expended in identifying natural enemies of tea pests that include predators, parasitoids, bacteria, viruses, and fungi (Ye et al., 2014). Entomopathogenic microbes are effective against the tea green leafhopper, Empoasca onukii Matsuda, the tea mosquito bug, Helopeltis theivora Water, the red spider mite, Oligonychus coffeae Nietner, among many others (Idris et al., 2020). In particular, beneficial fungi, including various Trichoderma species, and the entomopathogenic fungi Beauveria bassiana and Metarhizium anisopliae, have shown potential for control of tea mites and termites that attack tea, i.e., the live-wood eating termite, Microcerotermes beesoni Snyder, and other insect pests (Kumhar et al., 2020; Deka et al., 2021). However, infestation by the tea geometrid, Ectropis obliqua, remains difficult to control, with current pest management strategies broadly applying large doses of chemical pesticides. This is increasingly becoming problematic due to adverse ecological and human health effects of these chemicals and the potential for the development of resistance to such chemical agents, as immune defense and detoxification mechanisms in E. obliqua to certain pesticides, e.g., pyrethroids, have been shown to be robust (Yin et al., 2021). In addition, the desire for organic tea limits options for these growers. Synthetic volatile attractants for male moths have been developed, although the extent to which these are effective in controlling insect pests in the field remains to be determined since these could likely be used as part of larger control strategies (Sun et al., 2016).
Entomopathogenic fungi represent naturally occurring biocontrol agents which are increasingly easier to manufacture, offer convenient formulation, and can even help promote plant health via associations with plant roots and other structures (Dara, 2019). Thus, the application of these fungi can offer safer and more cost-effective alternatives and/or can be used to help decrease reliance on more toxic chemical agents. Entomopathogenic fungi have successfully been used for controlling some tea pests, although more efficacious isolates would increase this potential (Yang et al., 2022). Metarhizium spp. are one of the most widely studied and applied insect fungal pathogens and have been used to suppress multiple pests of different orders in diverse environments (Fernández-Bravo et al., 2021; Sharma et al., 2023). Identification of fungal isolates from specific insect hosts offers a means for identifying strains particularly virulent toward the desired insect target and represents the initial work required for subsequent selection and application in pest biological control/integrated pest management (IPM) programs. Here, after screening and isolation from insect cadavers on tea plants and from tea-growing region soils, we report the identification of four new record species of Metarhizium in China. Our data show previously unreported species of Metarhizium that offer a reservoir for discovery and potentially more directed application. In support of this idea, from the cohort characterized, we have identified a highly virulent isolate of M. pingshaense toward E. obliqua. Previous records of Metarhizium species have described M. anisopliae, M. brunneum, M. lepidiotae, M. majus, M. pinghaense, and M. robertsii in China (Xu et al., 2016). Earlier reports with M. pingshaense strains, particular, have shown them capable of infecting and resulting in significant mortalities toward the rice leaf folder, Cnaphalocrocis medinalis (Kirubakaran et al., 2018), the subterranean termite, Odontotermes obesus (Francis, 2019), various mosquitoes species (Lovett et al., 2019), and the yellow peach moth, Conogethes punctiferalis (Senthil Kumar et al., 2021); however, our isolate appears to be an excellent pathogen of E. obliqua. Characterization of morphological parameters including colony characteristics, shape, and size of the conidiophores, phialides, and conidia is generally employed to determine the identification of (insect pathogenic) fungal species (Mongkolsamrit et al., 2020), and the morphological traits of the fungal isolates reported here accorded well with previous depictions for each respective species of Metarhizium reported (Chen et al., 2021; Fernández-Bravo et al., 2021; Wei et al., 2022). To further confirm strain identities, we used molecular phylogenetic analyses based on multi-gene sequences of the TEF and RPB1genes, which have been shown to afford higher level discrimination than ITS, LSU, and/or tubulin sequences for this genus, allowing for species assignment to our isolates (Kepler et al., 2014; Senthil Kumar et al., 2021; Paolo Barzanti et al., 2023).
In laboratory bioassays, the various fungi were found to cause 40–93% mortality toward E. obliqua, when tested at a concentration of 1 × 108 conidia/mL indicating their different infective potentials and virulence against these insects as compared with a currently commercialized strain M. anisopliae CQMa421 which showed 79% mortality at the same concentration. Infected insects displayed signs of mycosis and copious sporulation 3–5 days post-mortem on host cadavers. High levels of sporulation produced on cadavers are a prominent feature of a successful biological control agent and can facilitate fungal spread under field conditions leading to epizootic infection and more effective control (Senthil Kumar et al., 2021). The median lethal concentration to kill 50% of infected insects (LC50) of the isolated highly virulent M. pingshaense MaFZ-13 strain against E. obliqua was calculated to be 9.6 × 104 conidia/mL, and the mean lethal time to kill 50% of target hosts (LT50) values was between 4.3 and 5.3 days. For other insects, previous findings using (different isolates of) M. pingshaense had described LC50 values ranging from 2.3 × 105 to 2.5 × 106 conidia/mL and LT50 values in the range of 4.7–6.4 days against late instar larvae of the yellow peach moth, Conogethes punctiferalis (Senthil Kumar et al., 2021), and 3.9–4.4 days against fourth instar larvae of rice leaf roller, C. medinalis (Kirubakaran et al., 2018). Thus, our data show high relative efficacy vs. E. obliqua. Greenhouse experiments were used to validate the laboratory bioassays as these sometimes do not equate to good control in field experiments. These data show that M. pingshaense MaFZ-13 was effective under these conditions and that larger scale field experiments are warranted. In addition, future experiments combining this strain with low doses of chemical pesticides and/or other methods (trapping) could result in robust IPM management in tea crops. These data indicate that we have identified a potential biocontrol agent for E. obliqua, an important pest of many plants throughout the world. Additional testing of the potential synergistic effects of M. pingshaense on plant growth, as this fungus is associated with the plant rhizosphere (Fernández-Bravo et al., 2021), is also warranted.
Data availability statement
The datasets presented in this study can be found in online repositories. The names of the repository/repositories and accession number(s) can be found in the article/Supplementary material.
Author contributions
CW, YC, and JQ: conceptualization. CW and JZ: methodology and visualization. CW, JZ, and YL: software. HP, JL, and SL: validation. PL and MZ: formal analysis. CW, JZ, and YC: investigation. XH, SC, and JQ: resources. XG: data curation. JZ and JQ: writing—original draft preparation. NK and JQ: writing—reviewing and editing. JQ: supervision, project administration, and funding acquisition. All authors contributed to the manuscript revision, and read and approved the submitted version.
Funding
This research was funded by the National Key R&D Program of China (no. 2017YFE0122000), the National Natural Science Foundation of China (no. U1803232, 32270029, and 31670026), a Key Project from the Fujian Provincial Department of Science and Technology (no. 2020N5005) and the Young and Middle-aged Teacher Education Research Project of Fujian Province (no. JAT210075), and NK was supported in part by USDA-NIFA grant 2019-05150.
Conflict of interest
The authors declare that the research was conducted in the absence of any commercial or financial relationships that could be construed as a potential conflict of interest.
Publisher's note
All claims expressed in this article are solely those of the authors and do not necessarily represent those of their affiliated organizations, or those of the publisher, the editors and the reviewers. Any product that may be evaluated in this article, or claim that may be made by its manufacturer, is not guaranteed or endorsed by the publisher.
Supplementary material
The Supplementary Material for this article can be found online at: https://www.frontiersin.org/articles/10.3389/fmicb.2023.1164511/full#supplementary-material
References
Beloti, V. H., Alves, G. R., Araújo, D. F., Picoli, M. M., Moral Rde, A., Demétrio, C. G., et al. (2015). Lethal and sublethal effects of insecticides used on citrus, on the ectoparasitoid Tamarixia radiata. PLoS ONE 10, e0132128. doi: 10.1371/journal.pone.0132128
Chen, W., Xie, W, Cai, W, Thaochan, N., and Hu, Q. (2021). Entomopathogenic fungi biodiversity in the soil of three provinces located in Southwest China and first approach to evaluate their biocontrol potential. J. Fungi. 7, 984. doi: 10.3390/jof7110984
Dara, S. K. (2019). Non-entomopathogenic roles of entomopathogenic fungi in promoting plant health and growth. Insects10, 277. doi: 10.3390/insects10090277
Deka, B., Babu, A., Peter, A. J., Kumhar, K. C., Sarkar, S., Rajbongshi, H., et al. (2021). Potential of the entomopathogenic fungus, Metarhizium anisopliae s.l. in controlling live-wood eating termite, Microtermes obesi (Holmgren) (Blattodea: Termitidae) infesting tea crop. Egypt. J. Biol. Pest Control 31, 132. doi: 10.1186/s41938-021-00477-4
Fernández-Bravo, M., Gschwend, F., Mayerhofer, J., Hug, A., Widmer, F., and Enkerli, J. (2021). Land-use type drives soil population structures of the entomopathogenic fungal genus Metarhizium. Microorganisms 9, 1380. doi: 10.3390/microorganisms9071380
Francis, J. R. (2019). Biocontrol potential and genetic diversity of Metarhizium anisopliae lineage in agricultural habitats. J. Appl. Microbiol. 127, 556–564. doi: 10.1111/jam.14328
Gao, T., Wang, Z., Huang, Y., Keyhani, N. O., and Huang, Z. (2017). Lack of resistance development in Bemisia tabaci to Isaria fumosorosea after multiple generations of selection. Sci. Rep. 7, 42727. doi: 10.1038/srep42727
Glare, T., Caradus, J., Gelernter, W., Jackson, T., Keyhani, N. O., Köhl, J., et al. (2012). Have biopesticides come of age? Trends Biotechnol. 30, 250–258. doi: 10.1016/j.tibtech.2012.01.003
Han, P., Han, J., Zhang, M., Fan, J., Gong, Q., Ma, E., et al. (2020). 20-Hydroxyecdysone enhances Immulectin-1 mediated immune response against entomogenous fungus in Locusta migratoria. Pest Manag. Sci. 76, 304–313. doi: 10.1002/ps.5515
Idris, A. L., Fan, X., Muhammad, M. H., Guo, Y., Guan, X., and Huang, T. (2020). Ecologically controlling insect and mite pests of tea plants with microbial pesticides: a review. Arch. Microbiol. 202, 1275–1284. doi: 10.1007/s00203-020-01862-7
Im, Y., Park, S. E., Lee, S. Y., Kim, J. C., and Kim, J. S. (2022). Early-stage defense mechanism of the cotton aphid Aphis gossypii against infection with the insect-killing fungus Beauveria bassiana JEF-544. Front. Immunol. 13, 907088. doi: 10.3389/fimmu.2022.907088
Kamga, S. F., Ndjomatchoua, F. T., Guimapi, R. A., Klingen, I., Tchawoua, C., Hjelkrem, A. R., et al. (2022). The effect of climate variability in the efficacy of the entomopathogenic fungus Metarhizium acridum against the desert locust Schistocerca gregaria. Sci. Rep. 12, 7535. doi: 10.1038/s41598-022-11424-0
Kepler, R. M., Humber, R. A., Bischoff, J. F., and Rehner, S. A. (2014). Clariffcation of generic and species boundaries for Metarhizium and related fungi through multigene phylogenetics. Mycologia 106, 811–829. doi: 10.3852/13-319
Kirubakaran, S. A., Abdel-Megeed, A., and Senthil-Nathan, S. (2018). Virulence of selected indigenous Metarhizium pingshaense (Ascomycota: Hypocreales) isolates against the rice leaffolder, Cnaphalocrocis medinalis (Guenèe) (Lepidoptera: Pyralidae). Physiol. Mol. Plant Pathol. 101, 105–115. doi: 10.1016/j.pmpp.2017.06.004
Kumar, S., Stecher, G., Li, M., Knyaz, C., and Tamura, K. (2018). MEGAX: molecular evolutionary genetics analysis across computing platforms. Mol. Biol. Evol. 35, 1547–1549. doi: 10.1093/molbev/msy096
Kumhar, K. C., Babu, A., Arulmarianathan, J. P., Deka, B., Bordoloi, M., Rajbongshi, H., et al. (2020). Role of beneficial fungi in managing diseases and insect pests of tea plantation. Egypt. J. Biol. Pest Control 30, 78. doi: 10.1186/s41938-020-00270-9
Li, Z. Q., Cai, X. M., Luo, Z. X., Bian, L., Xin, Z. J., Liu, Y., et al. (2019). Geographical distribution of Ectropis grisescens (Lepidoptera: Geometridae) and Ectropis obliqua in China and description of an efficient identification Method. J. Econ. Entomol. 112, 277–283. doi: 10.1093/jee/toy358
Lian, T., Qiu, H. L., Qin, C. S., Fox, E. G. P., Zhao, D. Y., Fang, T. S., et al. (2021). Metarhizium entomopathogenic fungi against the beetle Brontispa longissima (Coleoptera: Hispidae): isolation and species identification. Environ. Entomol. 50, 160–166. doi: 10.1093/ee/nvaa156
Lovett, B., Bilgo, E., Millogo, S. A., Ouattarra, A. K., Sare, I., Gnambani, E. J., et al. (2019). Transgenic Metarhizium rapidly kills mosquitoes in a malaria-endemic region of Burkina Faso. Science 364, 894–897. doi: 10.1126/science.aaw8737
Lu, X., Wang, B., Cai, X., Chen, S., Chen, Z., and Xin, Z. (2020). Feeding on tea GH19 chitinase enhances tea defense responses induced by regurgitant derived from Ectropis grisescens. Physiol. Plant. 169, 529–543. doi: 10.1111/ppl.13094
Ma, X. C., Shang, J. Y., Yang, Z. N., Bao, Y. Y., Xiao, Q., and Zhang, C. X. (2007). Genome sequence and organization of a nucleopolyhedrovirus that infects the tea looper caterpillar, Ectropis obliqua. Virology 360, 235–246. doi: 10.1016/j.virol.2006.10.024
Mahato, D., and Paikaray, N. (2021). Enotomopathogenic fungi: an efficient biological control agent for insects inhabitants management. Biotica Res. Today. 3, 595–597.
Mongkolsamrit, S., Khonsanit, A., Thanakitpipattana, D., Tasanathai, K., Noisripoom, W., Lamlertthon, S., et al. (2020). Revisiting Metarhizium and the description of new species from Thailand. Stud. Mycol. 95, 171–251. doi: 10.1016/j.simyco.2020.04.001
Ortiz-Urquiza, A., and Keyhani, N. O. (2013). Action on the surface: entomopathogenic fungi versus the insect cuticle. Insects 4, 357–374. doi: 10.3390/insects4030357
Ortiz-Urquiza, A., Luo, Z., and Keyhani, N. O. (2015). Improving mycoinsecticides for insect biological control. Appl. Microbiol. Biotechnol. 99, 1057–1068. doi: 10.1007/s00253-014-6270-x
Paolo Barzanti, G., Enkerli, J., Benvenuti, C., Strangi, A., Mazza, G., Torrini, G., et al. (2023). Genetic variability of Metarhizium isolates from the Ticino Valley Natural Park (Northern Italy) as a possible microbiological resource for the management of Popillia japonica. J. Invertebr. Pathol. 197, 107891. doi: 10.1016/j.jip.2023.107891
Portela, A. R., Hernandez, J. M., Bandeira, R. S., Junior, E. C. S., de Melo, T. C., Lucena, M. S. S., et al. (2021). Retrospective molecular analysis of norovirus recombinant strains in the amazon region, Brazil. Infect. Genet. Evol. 96, 105130. doi: 10.1016/j.meegid.2021.105130
Senthil Kumar, C. M., Jacob, T. K., Devasahayam, S., Geethu, C., and Hariharan, V. (2021). Characterization and biocontrol potential of a naturally occurring isolate of Metarhizium pingshaense infecting Conogethes punctiferalis. Microbiol. Res. 243, 126645. doi: 10.1016/j.micres.2020.126645
Sharma, A., Sharma, S., and Yadav, P. K. (2023). Entomopathogenic fungi and their relevance in sustainable agriculture: a review. Cogent Food Agr. 9, 2180857. doi: 10.1080/23311932.2023.2180857
Soh, L. S., and Veera Singham, G. (2021). Cuticle thickening associated with fenitrothion and imidacloprid resistance and influence of voltage-gated sodium channel mutations on pyrethroid resistance in the tropical bed bug, Cimex hemipterus. Pest Manag. Sci. 77, 5202–5212. doi: 10.1002/ps.6561
St Leger, R. J., and Wang, J. B. (2020). Metarhizium: jack of all trades, master of many. Open Biol. 10, 200307. doi: 10.1098/rsob.200307
Sun, X. L., Li, X. W., Xin, Z. J., Han, J. J., Ran, W., and Lei, S. (2016). Development of synthetic volatile attractant for male Ectropis obliqua moths. J. Integr. Agric. 15, 1532–1539. doi: 10.1016/S2095-3119(15)61294-1
Tang, Q. Y., and Zhang, C. X. (2013). Data Processing System (DPS) software with experimental design, statistical analysis and data mining developed for use in entomological research. Insect Sci. 20, 254–260. doi: 10.1111/j.1744-7917.2012.01519.x
Wang, Z., Li, H., Zhou, X., Tang, M., Sun, L., Zhan, S., et al. (2020). Comparative characterization of microbiota between the sibling species of tea geometrid moth Ectropis obliqua Prout and E. grisescens Warren. Bull. Entomol. Res. 110, 684–693. doi: 10.1017/S0007485320000164
Wei, D. P., Gentekaki, E., Wanasinghe, D. N., Tang, S. M., and Hyde, K. D. (2022). Diversity, molecular dating and ancestral characters state reconstruction of entomopathogenic fungi in Hypocreales. Mycosphere 13, 281–351. doi: 10.5943/mycosphere/si/1f/8
Wei, D. P., Wanasinghe, D. N., Hyde, K. D., Mortimer, P. E., Xu, J., Xiao, Y. P., et al. (2019). The genus Simplicillium. MycoKeys 60, 69–92. doi: 10.3897/mycokeys.60.38040
Xu, Y. J., Luo, F., Li, B., Shang, Y., and Wang, C. (2016). Metabolic conservation and diversification of Metarhizium species correlate with fungal host-specificity. Front. Microbiol. 7, 2020. doi: 10.3389/fmicb.2016.02020
Yan, Y., Zhang, Y., Tu, X., Wang, Q., Li, Y., Li, H., et al. (2020). Functional characterization of a binding protein for Type-II sex pheromones in the tea geometrid moth Ectropis obliqua Prout. Pestic. Biochem. Physiol. 165, 104542. doi: 10.1016/j.pestbp.2020.02.008
Yang, F. Y., Wu, Y. K., Dong, F., Tu, J., Li, X. F., Dong, Y., et al. (2022). Current status and prospect of entomopathogenic fungi for controlling insect and mite pests in tea plantations. J. Appl. Entomol. 146, 1041–1051. doi: 10.1111/jen.13080
Yang, Y. Q., Gao, X. H., Zhang, Y. Z., Zhang, L. W., and Wan, X. C. (2011). Comparative mating strategies of male and female Ectropis oblique (Lepidoptera: Geometridae). Florida Entomol. 94, 9–14. doi: 10.1653/024.094.0102
Ye, G. Y., Xiao, Q., Chen, M., Chen, X. X., Yuan, Z. J., Stanley, D. W., et al. (2014). Tea: biological control of insect and mite pests in China. Biol. Control 68, 73–91. doi: 10.1016/j.biocontrol.2013.06.013
Yin, H., Fu, Z. Z., Yang, X. X., Zhou, Y. Q, Mao, X. F., Liu, Z.Y., et al. (2021). Functional annotation of Ectropis obliqua transcriptome in the treatment of pyrethroid insecticides. Meta Gene 28, 100860. doi: 10.1016/j.mgene.2021.100860
Zeng, C., Wu, L., Zhao, Y., Yun, Y., and Peng, Y. (2018). Tea saponin reduces the damage of Ectropis obliqua to tea crops, and exerts reduced effects on the spiders Ebrechtella tricuspidata and Evarcha albaria compared to chemical insecticides. PeerJ 6, e4534. doi: 10.7717/peerj.4534
Zhang, S. Q., Feng, B. W., Zhang, J., Imboma, T., and Chen, L. L. (2020). Research progress on green control techniques of Ectropis grisescens Warren and Ectropis obliqua Prout. J. Environ. Entomol. 42, 1121–1138. doi: 10.3969/j.issn.1674-0858.2020.05.12
Zhang, X., Ran, W., Li, X., Zhang, J., Ye, M., Lin, S., et al. (2022). Exogenous application of gallic acid induces the direct defense of tea plant against Ectropis obliqua caterpillars. Front. Plant Sci. 13, 833489. doi: 10.3389/fpls.2022.833489
Keywords: Ascomycota, entomopathogenic fungus, Metarhizium, Ectropis obliqua, biocontrol potential, mycoinsecticide
Citation: Zhao J, Chen Y, Keyhani NO, Wang C, Li Y, Pu H, Li J, Liu S, Lai P, Zhu M, He X, Cai S, Guan X and Qiu J (2023) Isolation of a highly virulent Metarhizium strain targeting the tea pest, Ectropis obliqua. Front. Microbiol. 14:1164511. doi: 10.3389/fmicb.2023.1164511
Received: 12 February 2023; Accepted: 18 April 2023;
Published: 15 May 2023.
Edited by:
Ji-Chuan Kang, Guizhou University, ChinaReviewed by:
Jing Si, Beijing Forestry University, ChinaEustachio Tarasco, University of Bari Aldo Moro, Italy
Waqas Wakil, University of Agriculture, Faisalabad, Pakistan
Copyright © 2023 Zhao, Chen, Keyhani, Wang, Li, Pu, Li, Liu, Lai, Zhu, He, Cai, Guan and Qiu. This is an open-access article distributed under the terms of the Creative Commons Attribution License (CC BY). The use, distribution or reproduction in other forums is permitted, provided the original author(s) and the copyright owner(s) are credited and that the original publication in this journal is cited, in accordance with accepted academic practice. No use, distribution or reproduction is permitted which does not comply with these terms.
*Correspondence: Xiayu Guan, 47126940@qq.com; Junzhi Qiu, junzhiqiu@126.com
†These authors have contributed equally to this work