- 1Department of Environmental Microbiology and Biotechnology, Nicolaus Copernicus University in Toruń, Toruń, Poland
- 2Department of Aquatic Sciences and Assessment, Swedish University of Agricultural Sciences, Uppsala, Sweden
Inoculation with plant growth-promoting rhizobacteria (PGPR) is an eco-friendly sustainable strategy for improving crop productivity in diverse environments under different conditions. Our earlier study demonstrated that Pseudomonas sivasensis 2RO45 significantly stimulated canola (Brassica napus L. var. napus) growth. The aim of the present study was to investigate the structural and functional dynamics in the canola rhizosphere microbiome after inoculation with PGPR P. sivasensis 2RO45. The results based on alpha diversity metrics showed that P. sivasensis 2RO45 did not significantly alter the diversity of the native soil microbiota. However, the introduced strain modified the taxonomic structure of microbial communities, increasing the abundance of plant beneficial microorganisms, e.g., bacteria affiliated with families Comamonadaceae, Vicinamibacteraceae, genus Streptomyces, and fungi assigned to Nectriaceae, Didymellaceae, Exophiala, Cyphellophora vermispora, and Mortierella minutissima. The analysis of community level physiological profiling (CLPP) revealed that microbial communities in the P. sivasensis 2RO45 treated canola rhizospheres were more metabolically active than those in the non-treated canola rhizosphere. Four carbon sources (phenols, polymers, carboxylic acids, and amino acids) were better metabolized by the microbial communities from the rhizosphere of plants inoculated with the P. sivasensis 2RO45 than non-inoculated canola rhizospheres. Based on the community-level physiological profiles, the functional diversity of the rhizosphere microbiome was altered by the P. sivasensis 2RO45 inoculation. Substrate utilization Shannon diversity (H) index and evenness (E) index were significantly increased in the treated canola plants. The study provides new insight into PGPR-canola interactions for sustainable agriculture development.
1. Introduction
The rhizosphere is a hotspot around the roots where numerous important processes related to the nutrition, growth, and fitness of plants occur (Sugiyama et al., 2014; Zuluaga et al., 2021). Rhizosphere microorganisms, such as PGPR, are regarded as prominent components of sustainable agriculture due to their positive influence on plant growth through alleviation of biotic and abiotic stresses, providing nutrients and secretion of phytohormones (Zuluaga et al., 2021).
The native rhizosphere microbial communities are altered by numerous interactions, including interfering in root exudation patterns, exchange of genetic material, and transformation of nutrients. Rhizosphere microbiome may be also modified by PGPR inoculation (Bhattacharyya and Lee, 2016). Therefore, before the use of PGPR as bioinoculants in the field, it is necessary to determine the changes occurring in the resident soil microorganisms structure after PGPR inoculation. It was reported that PGPR inoculation can lead to transient or even permanent alterations in the abundance of microbial communities and their function, which can finally contribute to promoting plant growth and its fitness (Bhattacharyya and Lee, 2016; Kong and Liu, 2022).
Because rhizosphere microbial communities are important for plant growth promotion; an increasing number of studies pay more attention to the next generation sequencing (NGS) methods to better understand how PGPR inoculation affects the structure of rhizosphere microbiomes (Sugiyama et al., 2014). Moreover, it was reported that the effect of PGPR inocula on the functional diversity of the native rhizosphere microbial community should receive more attention (Di Salvo et al., 2018a). Some researchers analyzed the changes in microbial communities’ metabolic activity and function after PGPR inoculation using the community-level physiological profiling (CLPP) method. However, the analyses on different crops, such as rice, tomato, maize and wheat have been performed (Naiman et al., 2009; de Salamone et al., 2010; Di Salvo et al., 2018a; Zuluaga et al., 2021).
Our earlier results showed that the bacterization of canola (Brassica napus L. var. napus) seeds with Pseudomonas sivasensis 2RO45 significantly promoted growth of plant (Świątczak et al., unpublished). This study aimed to determine whether the bacterization of canola seeds with P. sivasensis 2RO45 alters the structural and functional diversity of microbial communities in the rhizosphere. The taxonomic structure changes in bacterial and fungal communities were evaluated using NGS, while the function of the microbial community was investigated by CLPP method using Biolog EcoPlates. The analyzes of interactions between canola plants and their associated microbiota after PGPR inoculation can provide new approaches for canola growth management.
2. Materials and methods
2.1. Rhizobacterium Pseudomonas sivasensis 2RO45
The Pseudomonas sivasensis 2RO45 was originally isolated from the canola (Brassica napus L. var. napus) rhizosphere taken from the field in Ostroda, Poland (53°41′38″N 19°57′58″E). The 2RO45 was selected based on its PGP traits, and its ability to promote canola growth under sterile and non-sterile conditions. The 2RO45 was able to produce indole-3-acetic acid (IAA), sequester siderophores, solubilize phosphates, and to produce 1-aminocyclopropane-1-carboxylic acid (ACC) deaminase.
2.2. Experimental design and rhizospheric soil sampling
Pseudomonas sivasensis 2RO45 cells were harvested in LB broth (10 ml) with 0.05 g carboxymethyl cellulose (CMC) to yield 108 colony forming units (CFU)/ml. Seeds of canola were sterilized by soaking for 30 min in 1% sodium hypochlorite, followed by three rinses with sterile distilled water and incubation with 2RO45 inoculum for 30 min in a shaker incubator. Control seeds were incubated in LB broth (10 ml) with 0.05 g CMC under the same conditions (Rudolph et al., 2015). Four seeds were sowed in pots containing soil taken from the field in Ostroda, Poland (53°41′38″N 19°57′58″E). The bacterized and non-bacterized rhizospheres samples (total of 24 samples) were collected from the canola roots by scraping the adhering soil after 0 (T0), 22 (T22), and 44 days (T44). Four replicate pots were maintained for each time-point (Supplementary Figure 1). The “time” was considered as a main factor for establishing the groups of samples, in which the changes in microbiota structure and function were evaluated.
2.3. Colonization efficiency of Pseudomonas sivasensis 2RO45
To test if P. sivasensis 2RO45 can colonize the canola rhizosphere, sterilized canola seeds were inoculated with 2RO45 (108 CFU/ml) under sterile soil conditions. Canola plants were maintained in a growth chamber in a day-night cycle of 16 h light (100 μmol/m2/s) and a temperature of 22°C. Root samples were collected after 0 (T0), 22 (T22), and 44 days (T44). The plant roots were vortexed for 30 min in PBS solution (Ke et al., 2019) and the number of P. sivasensis 2RO45 were counted on PCA medium after incubating at 28°C for 48 h.
2.4. DNA extraction and sequencing
Rhizosphere samples collected from the replicate pots of each treatment were pooled separately (Supplementary Figure 1; Bhattacharyya et al., 2018) and total genomic DNA was extracted from 0.25 g of fresh rhizosphere samples using a DNeasy Power Soil Kit (Qiagen, Hilden, Germany), following the manufacturer’s protocol. DNA concentration was determined using Qubit dsDNA HS Assay Kit (Thermo Fisher Scientific, Waltham, MA, USA). Then, each sample was sent for (NGS) at the University of Łódź (Biobank), Poland.
Bacterial and fungal communities were determined by the amplification of V3-V4 hyper-variable regions of the 16S rRNA gene and Internal Transcribed Spacer (ITS2), respectively. For bacteria, the following primers were used: 5′ CCTACGGGNGGCWGCAG (forward) and 5′ GACTACHVGGGTATCTAATCC (reverse) (Klindworth et al., 2013). For fungi, a forward primer was used: 5′GCATCGATGAAGAACGCAGC (ITS3, without overhangs) with the reverse primer 5′ TCCTCCGCTTATTGATATGC (ITS4, without overhangs) (White et al., 1990). The methodology for library preparation followed the protocol available from Illumina Support Center (ISC) with a slight modification (2 × Phanta Max Master Mix, Vazyme Biotech, Nanjing City, China was applied instead of Kapa Hifi Hot Start Ready mix). The products were verified after each PCR using the electrophoretic separation. Libraries were normalized based on band luminescence intensity on a 1.5% agarose gel and pooled. Sequencing was performed on a MiSeq (Illumina, San Diego, CA, USA) using MiSeq Reagent Kit v2 (500-cycles) 2 × 250 bps paired-end format.
2.5. Amplicon sequence analysis
In the present study, the merging of sequence sets was performed, and the analysis of 16S rRNA amplicons was carried out using mothur v1.44.3 (Schloss et al., 2009). The quality processing, taxonomic assignments, and operational taxonomic unit (OTU) picking were done according to the MiSeq SOP of mothur (Kozich et al., 2013) with a 97% similarity threshold. The ‘make.contigs’ command was executed with a deltaq value of 10, and the analysis excluded ambiguous base calls and reads shorter than 300 nt or longer than 500 nt. The end of the sequences was trimmed to remove the primers using trim.seqs (pdiffs = 2, checkorient = T). The pre.cluster command was utilized for denoising, and chimeric reads were filtered out using VSEARCH in mothur. The sequence set was further processed by discarding the singleton reads as per Kunin et al. (2010). The read alignment and taxonomic assignment were carried out using the ARB-SILVA SSU Ref NR 138 reference database (Quast et al., 2013) with a minimum bootstrap confidence score of 80. Reads assigned to non-primer-specific taxonomic groups (“Chloroplast,” “Mitochondria,” and “unknown”) were excluded from the dataset. Finally, a random subsampling was performed based on the sample with the lowest sequence number.
Fungal ITS analysis was performed through the following steps. The merging and quality filtering of sequences were carried out using mothur, as described for the 16S rRNA gene amplicons. The ITS2 region was extracted from the sequences with the ITSx 1.1-beta software (Bengtsson-Palme et al., 2013) based on the findings of Nilsson et al. (2010). Taxonomic assignment was performed using mothur’s classify.seqs (cutoff = 80) and the UNITE v8.3 database (Abarenkov et al., 2020) as reference. Sequences that were not assigned to any fungal phyla were discarded from the amplicon set with the remove.lineage (taxon = k__Fungi_unclassified) command of mothur. Finally, the clustering of ITS2 reads into OTUs was performed using VSEARCH with a 97% similarity threshold.
The sequences were uploaded to the GenBank-Sequence Read Archive under Bioproject PRJNA876229.
2.6. Functional potential of microbial communities
The effect of Pseudomonas sivasensis 2RO45 inoculation on the microbial community function and diversity in the canola rhizosphere were analyzed by the CLPP method using Biolog EcoPlates (Biolog Inc., Hayward, CA, USA). Rhizosphere samples (10 g) were collected after 44 days of P. sivasensis 2RO45 inoculation. Samples were added to 90 ml of 0.85% sterile saline solution and shaken for 30 min at 200 rpm (Sun et al., 2010). Samples were diluted to a 10–2 gradient and 150 μl suspension of the dilution obtained from each rhizosphere sample was applied to Biolog EcoPlates wells. The plates were incubated at 28°C and the optical density (OD) was measured at 595 nm using a microplate absorbance spectrophotometry (Multiskan EX, Thermofisher Scientific, Waltham, MA, USA) at 24 h intervals up to 7 days incubation. Three replicates were performed for each treatment.
The microbial activity was calculated by the average well color development (AWCD) using the following equation: AWCD = Σ (C–R)/31, where C is the absorbance value of each well, and R is the absorbance value of the control (Bhattacharyya and Lee, 2016). The 31 substrates were divided into six kinds of carbon sources: phenols (pyruvic acid methyl ester, glucose phosphate, glycerol phosphate), polymers (tween 40, tween 80, cyclodextrin), carbohydrates (cellobiose, lactose, b-methyl-d-glucoside, d-xylose, erythritol, mannitol), carboxylic acids (glucosaminic acid, b-galactonic acid, galacturonic acid, hydroxybenzoic acid, 4 hydroxybenzoic acid, hydroxybutyric acid, itaconic acid, keto butyric acid, malic acid), amino acids (L-arginine, asparagine, phenylalanine, serine, threonine. glycyl glutamic acid) and amines/amides (phenylethylamine, putrescine). The AWCD for each carbon substrate group was determined using the following formula: AWCD = Σ (C–R)/N, where C is the absorbance value of each well, R is the absorbance value of the control and N is the number of substrates in the category (Bhattacharyya and Lee, 2016; Zuluaga et al., 2021). The functional diversity of microbial communities was expressed as the substrate utilization Shannon diversity index (H), substrate utilization Shannon evenness index (E) and substrate utilization Simpson diversity index (D) (Ge et al., 2018; Koner et al., 2021). The absorbance values on the 7th day of incubation were used for the calculation of metabolic functional diversity indices.
2.7. Microbial community structure
Richness estimators and diversity indices were calculated with mothur. LEfSe (Linear discriminant analysis effect size) pipeline, available at http://huttenhower.sph.harvard.edu/galaxy/ was used to identify taxonomic bacterial and fungal groups that were differentially abundant (p < 0.05) in the untreated and Pseudomonas sivasensis 2RO45 treated plants at each time of the inoculation (T0, T22, and T44). This analysis was performed for the 50 most abundant OTUs.
2.8. Data analysis and statistics
Rarefaction curves were generated using phyloseq in R v 4.0.3. Differences in microbial alpha diversity [observed OTU richness, Shannon (H’), and Simpson index (1-D)] were estimated using Past v 3.08. Two different statistical analyses in terms of microbial alpha diversity were performed: (i) t-test for equal means to detect differences between non-treated and Pseudomonas sivasensis 2RO45 treated canola plants; (ii) one-way analysis of variance (ANOVA) followed by post-hoc test (Tukey’s HSD test; p-values ≤ 0.05) to evaluate differences in the rhizosphere of Pseudomonas sivasensis 2RO45 treated and untreated plants according to time. Normality and homogeneity of variance assumptions were performed by the Shapiro-Wilk and Levene’s tests. Principal coordinates analysis (PCoA) and analysis of similarities (ANOSIM) were performed using R (v 4.0.3).
3. Result and discussion
The bioinformatic data processing resulted in 2,198,786 and 2,121,080 high quality bacterial and fungal sequence reads, respectively. Datasets covered 16,443 bacterial and 2,760 fungal OTUs. The rarefaction curves indicated a high coverage of the estimated bacterial and fungal diversity in the canola rhizosphere samples (Supplementary Figure 2).
Different alpha biodiversity indices for bacteria, e.g., OTU richness, Shannon, and Inv Simpson significantly increased over time, both in non-treated and treated with Pseudomonas sivasensis 2RO45 rhizosphere samples (Supplementary Table 1). Whereas, fungal alpha-diversity based on the OTU richness and Shannon diversity index were the highest in non-treated samples on 44th day (Supplementary Table 2). Rhizosphere bacterial and fungal communities were altered by various environmental factors such as climate and soil properties (Li et al., 2021), as well as host factors such as plant genotype and plant age (Fazal et al., 2021). Our results indicated that microbial richness and diversity increase with time and plant development. Previous studies also confirmed that the abundance and structure of the soil microbial community can differ depending on the plant growth stage (Liu et al., 2015; Wang et al., 2017).
Plant growth-promoting rhizobacteria inoculants can be a suitable option to developing sustainable agriculture, reducing or even eliminating the use of agrochemicals without yield loss (Kong and Liu, 2022). Nonetheless, understanding the effect of PGPR inoculation on the structure and functional dynamics in the rhizosphere microbiome is essential for better exploitation of PGPR potential in improving plant health and fitness (Zuluaga et al., 2021; Kong and Liu, 2022). Therefore, we studied biodiversity of the microbial community in the canola rhizosphere after inoculation with Pseudomonas sivasensis 2RO45 which features the ability to improve canola growth.
There were no significant differences, given the alpha diversity metrics between non-treated and P. sivasensis 2RO45 treated canola plants (Figure 1), indicating that the 2RO45 strain did not significantly alter the diversity of the native soil microbiota. These results were also supported by PCoA (Figure 2) and ANOSIM (Table 1). No significant bacterial, archaeal, and diazotrophic enrichments were observed in the rhizosphere of maize (Zea mays L.) after Pseudomonas stutzeri A1501 inoculation (Ke et al., 2019). There are reports demonstrating that PGPR inoculation increases the microbial diversity in the rhizosphere, simultaneously promoting Kimchi cabbage (Brassica rapa L. ssp. pekinensis) growth (Yu and Lee, 2013; Bhattacharyya et al., 2018). However, if the PGPR inoculants are unable to survive in the competition with indigenous bacterial communities, minor or no changes would happen in terms of rhizosphere microbiome structure or diversity (Piromyou et al., 2011; Touceda-González et al., 2015). Although PGPR inoculation caused only minor changes in the rhizosphere microbial community, plant growth promotion has been observed (Piromyou et al., 2011; Touceda-González et al., 2015). Jiménez et al. (2020) showed that PGPR Pseudomonas fluorescens LBUM677 influences the rhizosphere microbiome of three different oilseed crops: canola (Brassica napus L.), soybean (Glycine max L.), and corn gromwell (Buglossoides arvensis L.). Interestingly, P. fluorescens LBUM677 inoculation decreased diversity of these rhizosphere microbiomes (Jiménez et al., 2020). According to Kong and Liu (2022) the effectiveness of inoculated PGPR is associated with their ability to being efficient rhizosphere colonizers. Our results showed that based on the relative abundance of the Pseudomonas phylotype (OTU00038) in the total bacterial community (Supplementary Figure 3) one can conclude that some Pseudomonas bacteria were already present in the soil before the treatment, and the inoculation of Pseudomonas sivasensis 2RO45 did not necessarily cause the substantial proliferation of this bacteria in the soil. Moreover, colonization of the inoculated Pseudomonas sivasensis 2RO45 in the rhizosphere in sterile conditions, measured as CFU was determined. The results showed that the strain was able to survive up to 44 days (Supplementary Table 3). The viability of the strain ranged from 6.6 × 106 CFU/ml at T0 to 5.5 × 106 CFU/ml at T44. Nevertheless, according to Chen et al. (2022), neither the colonization of roots by PGPR inoculants, nor modifications in the rhizosphere microbiome were necessary for the plant growth promotion process. The authors demonstrated that PGPR-induced DNA methylation modifications in roots mediated the long-term impact on plant growth promotion (Chen et al., 2022).
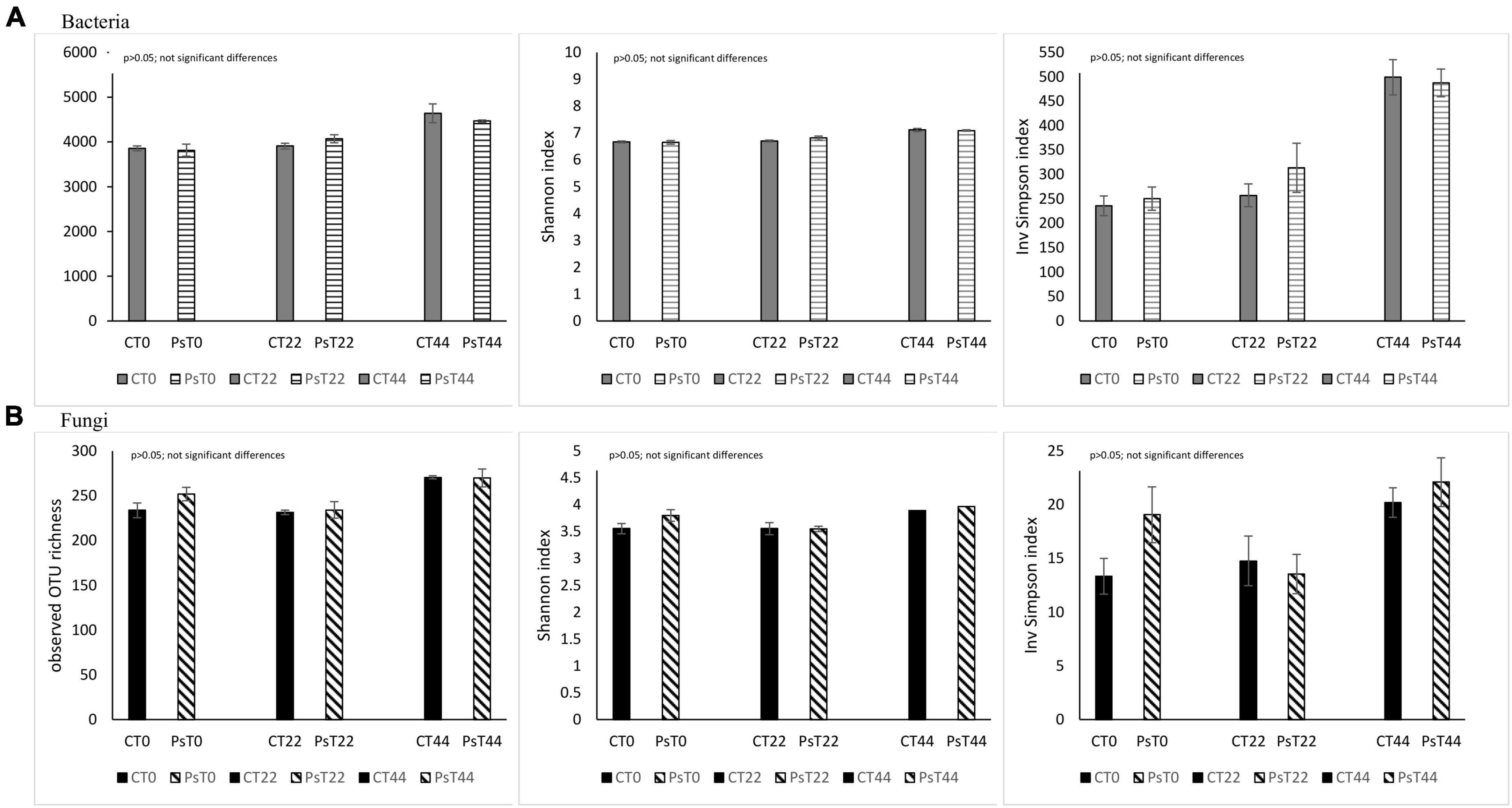
Figure 1. OTU richness and diversity indices (Shannon H’ and Inv Simpson) for the bacterial (A) and fungal (B) communities in Pseudomonas sivasensis 2RO45 treated canola rhizospheres and untreated rhizospheres samples according to treatment based on NGS sequencing; vertical bars represent standard deviation (n = 3).
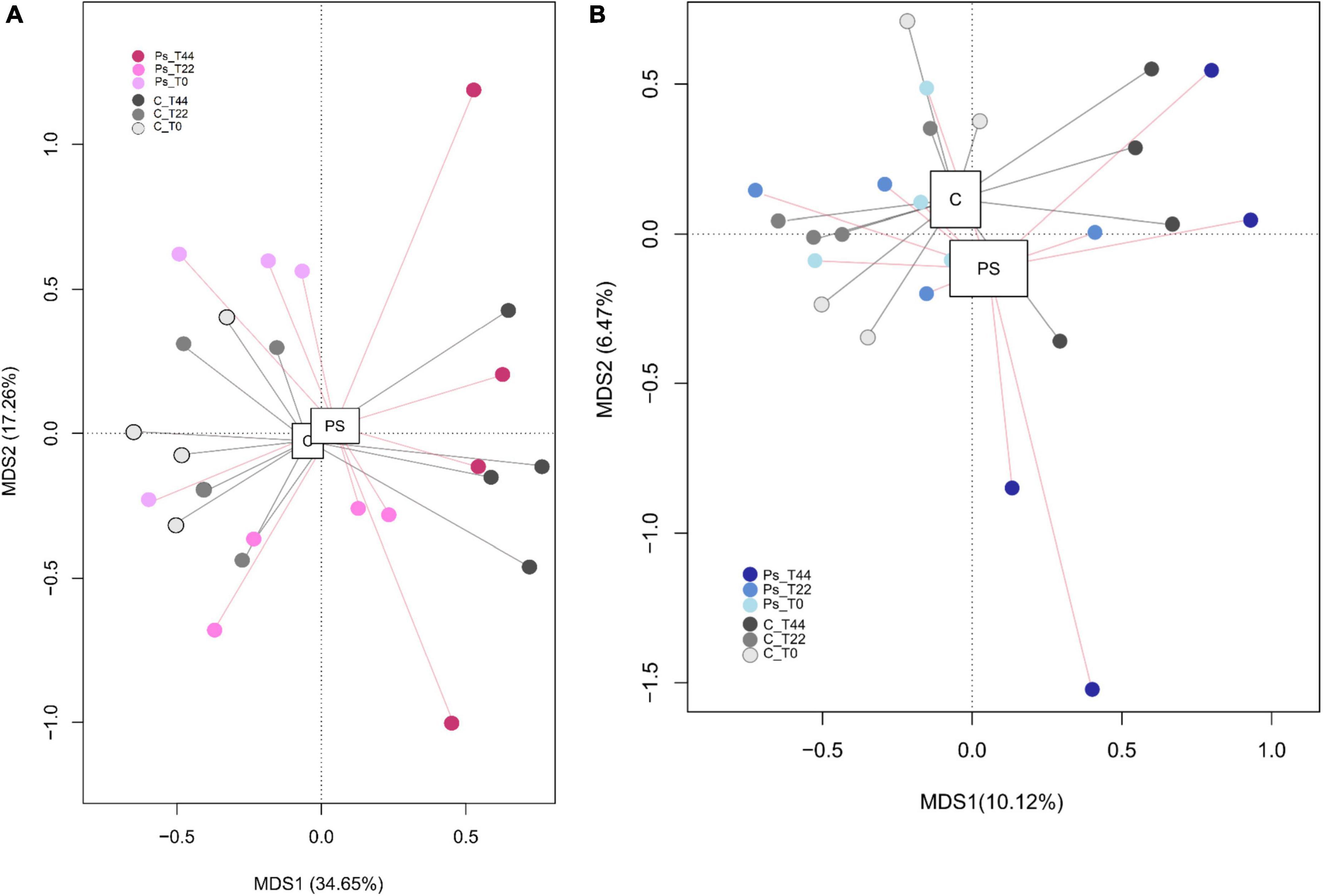
Figure 2. Principal coordinates analysis of bacterial (A) and fungal (B) distributions in canola plants inoculated with Pseudomonas sivasensis 2RO45 (PS) or uninoculated (C); T0, T22, and T44–time after inoculation in days.
Pseudomonas sivasensis 2RO45 treatment did not alter the overall microbial diversity, but changed the proportions of some bacterial (Figure 3) and fungal (Figure 4) taxa. The introduced strain modified microbial communities, particularly increasing the abundance of microorganisms beneficial for a plant growth. Initially at T0, P. sivasensis, 2RO45 increased the abundance of genus Streptomyces, then at T22 bacterial families Comamonadaceae and Vicinamibacteraceae were more abundant in the P. sivasensis 2RO45 treated soil. Whereas, at T44 P. sivasensis 2RO45 strain did not cause any changes in the bacterial community structure. Numerous studies reported that Streptomyces has beneficial associations with plants, improving their growth and protecting them against bacterial and fungal diseases through the production of antibiotics and bioactive compounds (Amaresan et al., 2018; Suárez-Moreno et al., 2019; Vergnes et al., 2020; Le et al., 2021). Bacteria belonging to the Vicinamibacteraceae family are degraders of organic matter and chitin (Whitton et al., 2022), while members of the family Comamonadaceae are able to control Fusarium wilt disease by secreting more organic acids (Wen et al., 2020). The changes in taxonomic structure until 22 days after P. sivasensis 2RO45 bacterization indicated a transient and short-term perturbation in the taxonomic structure of bacterial communities. The results also indicate that the taxonomic structure of bacterial communities in young canola plants was more dynamically influenced by P. sivasensis 2RO45 bacterization compared to that in older plants. Similar results were obtained by Bhattacharyya and Lee (2016).
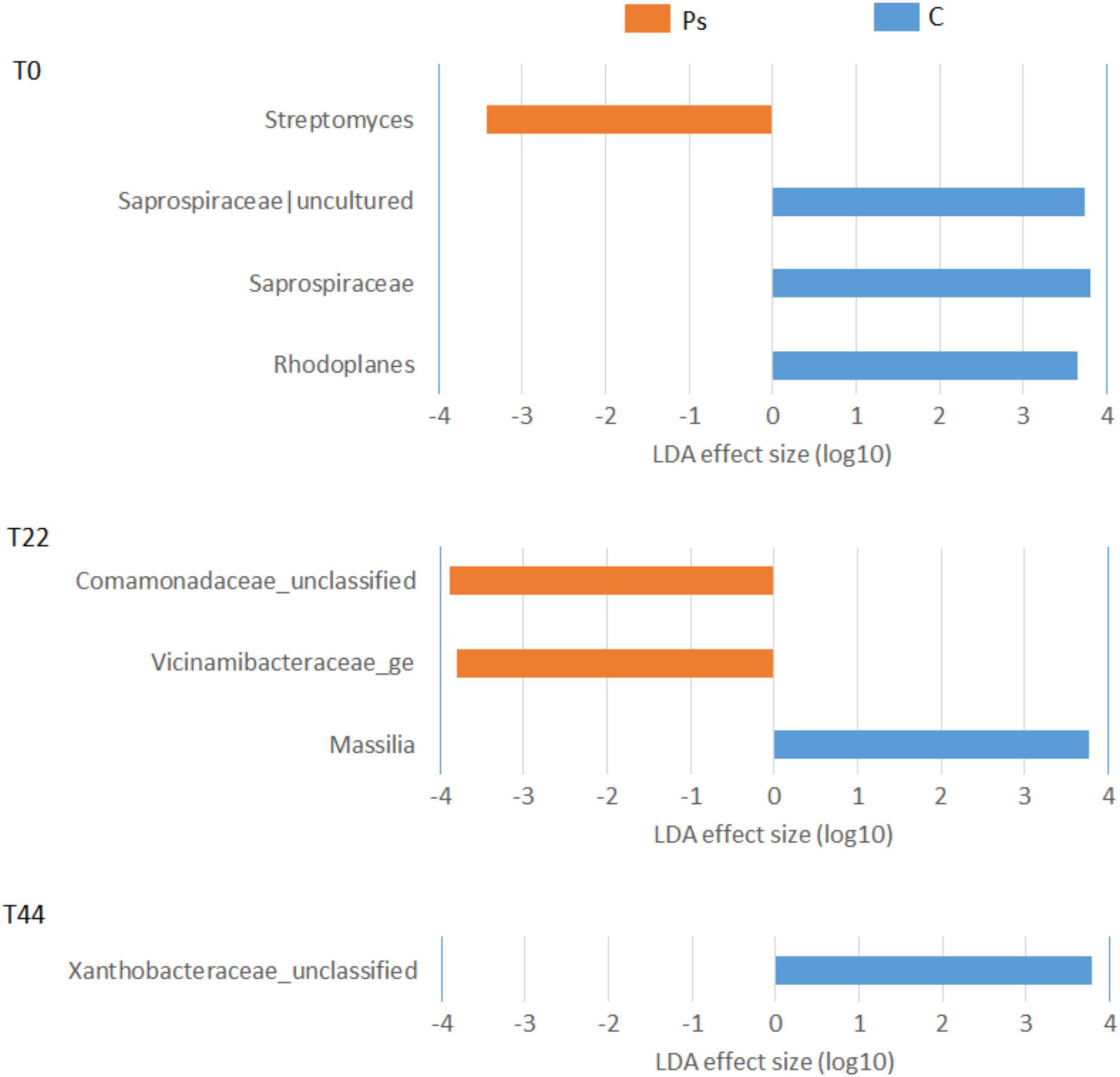
Figure 3. Linear discriminant analysis effect size (LEfSe) analysis showing bacterial taxonomy changes between canola plants inoculated with Pseudomonas sivasensis 2RO45 (PS) and uninoculated (C); T0, T22, and T44–time after inoculation in days.
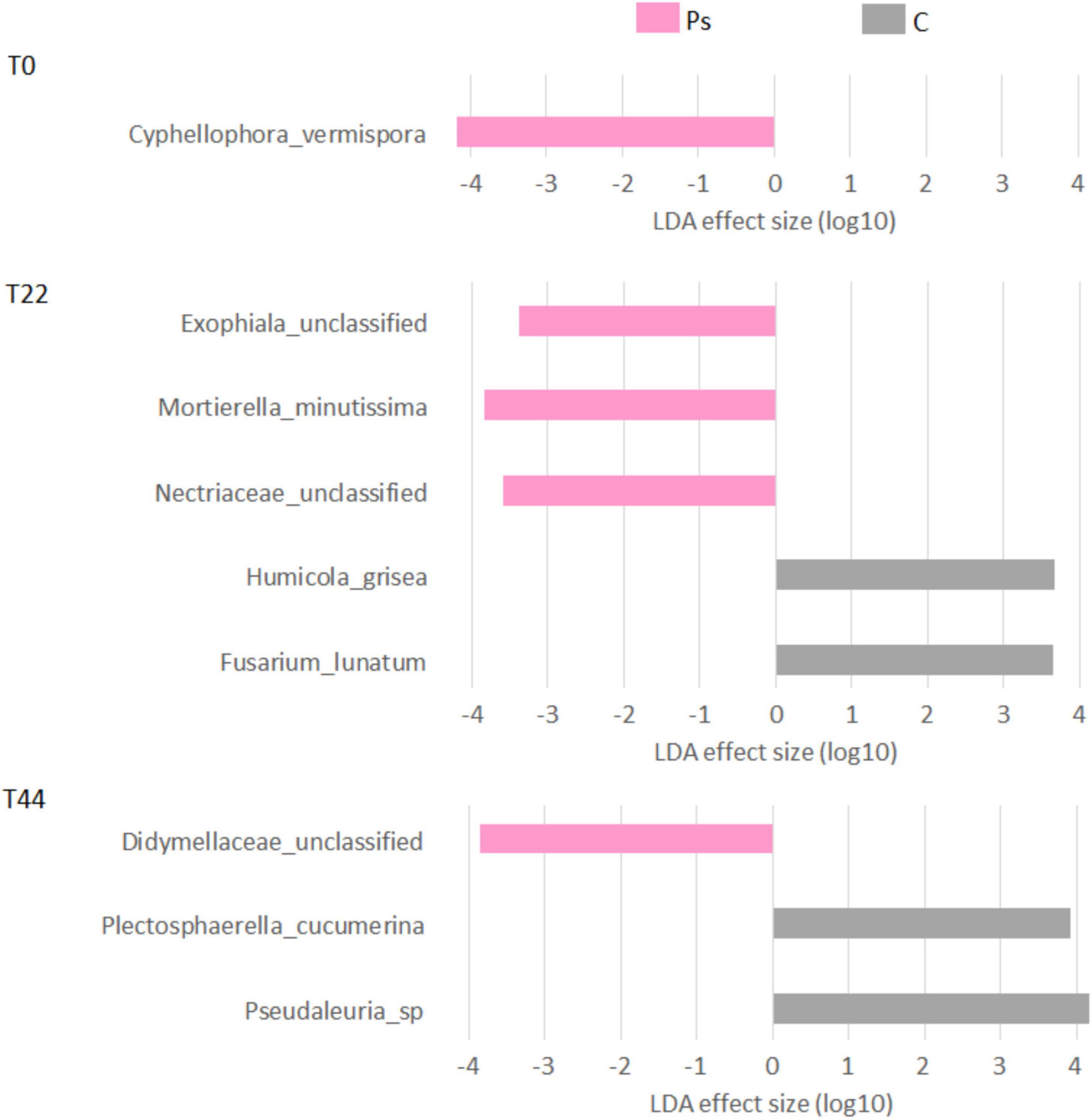
Figure 4. Linear discriminant analysis effect size (LEfSe) analysis showing fungal taxonomy changes between canola plants inoculated with Pseudomonas sivasensis 2RO45 (PS) and uninoculated (C); T0, T22, and T44–time after inoculation in days.
After P. sivasensis 2RO45 inoculation into the soil, the highest changes in fungal communities in comparison to the control were observed at T22. Initially at T0, Cyphellophora vermispora was more abundant in P. sivasensis 2RO45 treated soil, then at T22 P. sivasensis 2RO45 increased the abundance of the fungal family of Nectriaceae, genus Exophiala and species Mortierella minutissima. Whereas, at T44 P. sivasensis 2RO45 increased the abundance of the Didymellaceae family. Species of Didymellaceae were found as plant fungal pathogens causing fruit, leaf, stem, and root lesions on a wide variety of crops (Hou et al., 2020). However, Didymellaceae is the largest family within the order Pleosporales with more than 5 400 taxon names, including also saprobic, endophytic, and clinically relevant species (Hou et al., 2020; Yuan et al., 2021). Exophiala has been effectively used in a agricultural biotechnology. For instance, Exophiala pisciphila stimulated maize growth (Xu et al., 2020) and suppressed Fusarium-wilt disease in strawberries (Harsonowati et al., 2020), while Exophiala sp. promoted cucumber growth under abiotic stresses (Khan et al., 2011). Cyphellophora vermispora was isolated from a natural environment such as plant stems, roots, and leaves (Gao et al., 2015). Members of the Nectriaceae family have been reported as plant opportunistic pathogens; however, several species belonging to the Nectriaceae have been also used as biocontrol agents and biodegraders for developing sustainable agriculture (Lombard et al., 2015). The genus Mortierella includes numerous PGP fungi degrading biopolymers, e.g., M. minutissima isolated from the root surface had a strong chitinolytic activity (Ozimek and Hanaka, 2020).
The community-level physiological profiling (CLPP) using Biolog EcoPlates was estimated to analyze the changes in the canola rhizosphere metabolic profile and functional diversity in response to P. sivasensis 2RO45 introduction. The alterations in the metabolic profile of microbial communities from non-treated and P. sivasensis 2RO45 treated rhizospheres were analyzed on the 44th day. This time point was chosen to reveal if P. sivasensis 2RO45 inoculation has a long-term impact on the functional diversity of the canola rhizosphere microbiome.
The metabolic functional diversity indices, except substrate utilization Simpson index (D), had significant differences (p < 0.05) between non-treated and P. sivasensis 2RO45 treated canola rhizospheres (Table 2). Substrate utilization Shannon diversity (H) index and evenness (E) were the highest in canola plants inoculated with P. sivasensis 2RO45, indicating that the functional diversity of the canola rhizosphere microbial community was altered by bacterization. The changes in the metabolic profiles of soil microbial communities after PGPR introduction is in line with previous studies on plants, such as wheat (Di Salvo et al., 2018b), maize (Di Salvo et al., 2018a), tomato (Zuluaga et al., 2021), and rice (de Salamone et al., 2010).
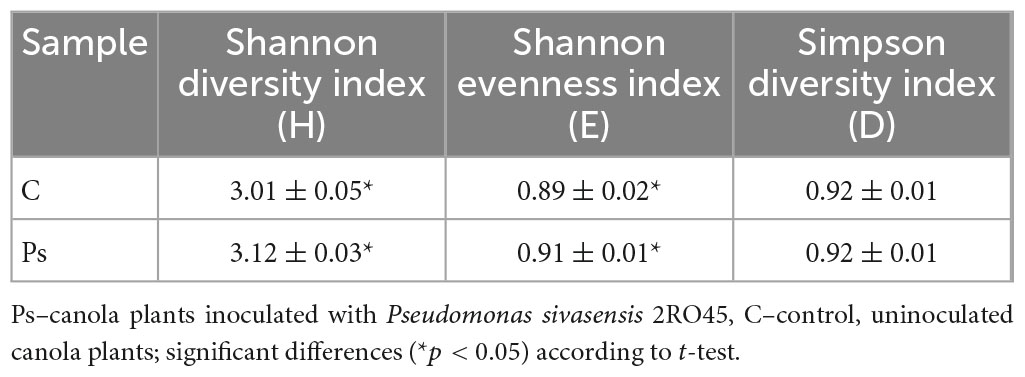
Table 2. Functional diversity indices based on Biolog EcoPlates results on the 7th day of incubation for the canola rhizosphere microbial communities.
The average well color development (AWCD) curve of all carbon sources was plotted to check the metabolic activity of microbial communities (Figure 5). The microbial communities of the P. sivasensis 2RO45 treated canola rhizospheres samples exhibited higher AWCD values than the control samples, suggesting that microbiota associated with P. sivasensis 2RO45 were more active in their use of different types of carbon substrates during cell growth than the indigenous microbiota and the overall metabolic activity was increased by bacterization. Furthermore, the microbial activity in the canola rhizospheres was calculated by AWCD for six categories of carbon sources: phenols, carbohydrates, amino acids, carboxylic acids, polymers, and amines (Figure 6). The microbial communities in the P. sivasensis 2RO45 treated canola rhizospheres samples were found to utilize phenols, polymers, carboxylic acids, and amino acids more intensively than those in the non-treated samples. Whereas, carbohydrates and amines were better metabolized by the rhizosphere community from non-treated canola plants. The results are similar to the findings reported by previous research, where the metabolic profile of microbial communities was modified due to PGPR bacterization (Bhattacharyya and Lee, 2016; Zuluaga et al., 2021). According to Bhattacharyya and Lee (2016), an increase in the utilization of amino acids and a reduction in the utilization of carbohydrates in the bacterized rhizosphere could have a beneficial effect on Kimchi cabbage growth. Whereas, Zuluaga et al. (2021) found that carbohydrates, amines, polymers, and phenolic compounds were the main carbon substrate groups that contribute to the rhizosphere microbiome function between rhizosphere plants inoculated with PGPR Pseudomonas sp. and non-inoculated plants. The authors suggested that rhizobacterial inoculants can modulate the rhizosphere microbiome function by affecting the root exudation profile, consequently interfering in the plant-soil feedback and the shaping of the plant-associated microbial communities (Zuluaga et al., 2021).
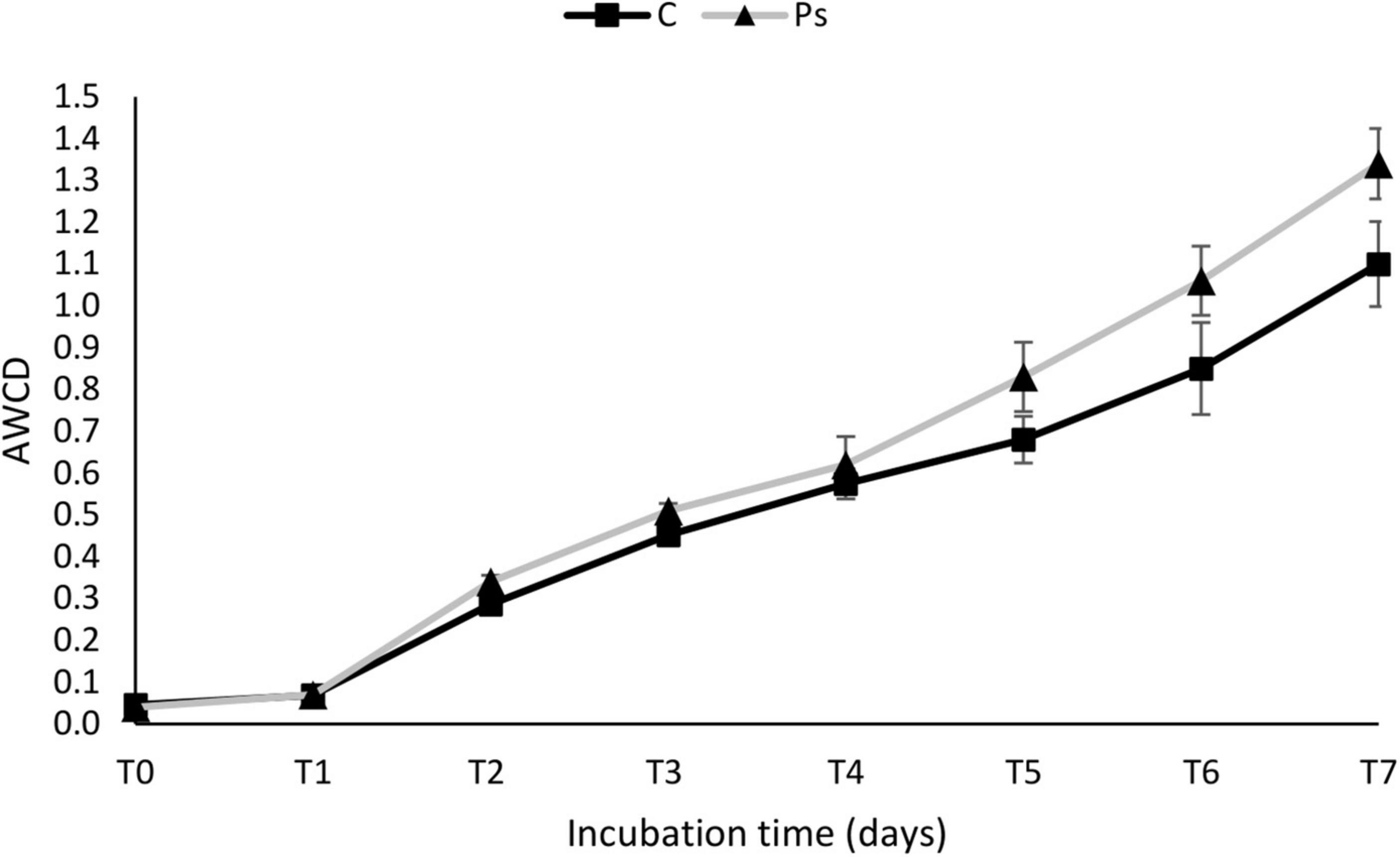
Figure 5. The average well color development (AWCD) of all carbon sources in Pseudomonas sivasensis 2RO45 treated and non-treated rhizosphere samples according to incubation time; vertical bars represent standard deviation (n = 3).
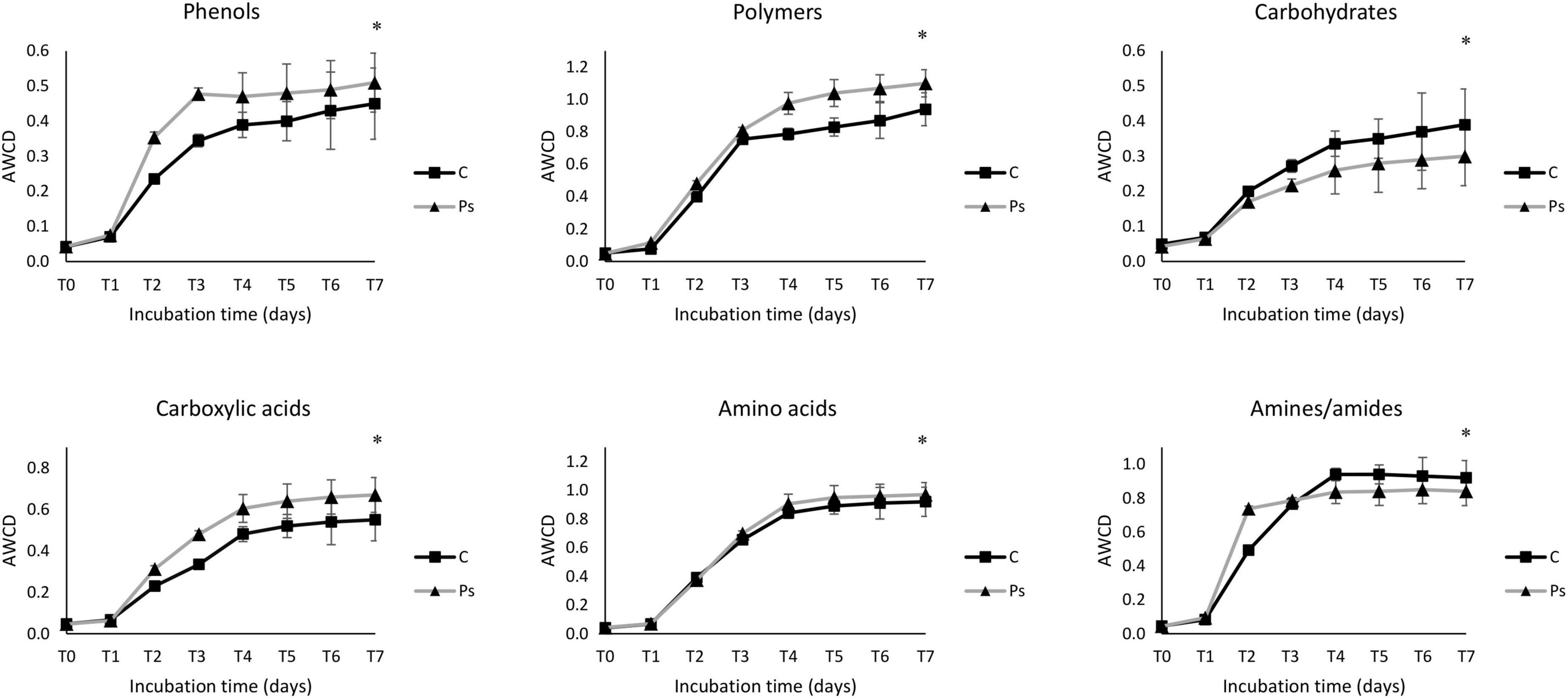
Figure 6. The average well color development (AWCD) of six carbon source groups, including phenols, polymers, carbohydrates, carboxylic acids, amino acids, and amines/amides in Pseudomonas sivasensis 2RO45 treated and non-treated rhizosphere samples; vertical bars represent standard deviation (n = 3); significant differences (*p < 0.05).
4. Conclusion
Plant growth-promoting rhizobacteria Pseudomonas sivasensis 2RO45 inoculation altered the taxonomic structure of canola rhizosphere microbial communities by increasing the abundance of plant beneficial microorganisms: bacteria affiliated with families Comamonadaceae, Vicinamibacteraceae, genus Streptomyces, and fungi assigned to the Nectriaceae, Didymellaceae, Exophiala, Cyphellophora vermispora, and Mortierella minutissima. Moreover, P. sivasensis 2RO45 induced perturbations in the rhizosphere microbiome by increasing metabolic activity and functional diversity of microbial communities. Phenols, polymers, carboxylic acids, and amino acids were the major classes of carbon substrates that contributed to the function of the rhizosphere microbiome after inoculation with P. sivasensis 2RO45. The results provide new insight and future perspectives into PGPR-canola interactions for sustainable agriculture development. The introduction of P. sivasensis 2RO45 is beneficial to development of a sustainable agriculture because it changes the native microbiota crucial for the proper functioning of the soil. The minor changes that are observed are positive, especially the increase of metabolic activity which may increase the possibility of detoxification of the environment (decomposition of phenols and polymers) or the elements circulation (e.g., carbon and nitrogen).
Data availability statement
The datasets presented in this study can be found in online repositories. The names of the repository/repositories and accession number(s) can be found in the article/Supplementary material.
Author contributions
JŚ wrote the original draft, made greenhouse experiment and sampling, made isolation of DNA, made community-level physiological profiles (CLPP) analysis using Biolog EcoPlates, conducted statistical analyses, and prepared the manuscript editorially. AK made rarefaction curves, LEfSe PCoA, and ANOSIM analysis, coordinated the study, and checked the validity of the original draft. AS carried out the sequence analysis. MS designed and coordinated the study and checked the validity of the original draft. All authors contributed to the article and approved the submitted version.
Funding
This research was supported by funds provided by Nicolaus Copernicus University (Toruń, Poland) to maintain research potential.
Conflict of interest
The authors declare that the research was conducted in the absence of any commercial or financial relationships that could be construed as a potential conflict of interest.
Publisher’s note
All claims expressed in this article are solely those of the authors and do not necessarily represent those of their affiliated organizations, or those of the publisher, the editors and the reviewers. Any product that may be evaluated in this article, or claim that may be made by its manufacturer, is not guaranteed or endorsed by the publisher.
Supplementary material
The Supplementary Material for this article can be found online at: https://www.frontiersin.org/articles/10.3389/fmicb.2023.1168907/full#supplementary-material
References
Abarenkov, K., Zirk, A., Piirmann, T., Pöhönen, R., Ivanov, F., Nilsson, R. H., et al. (2020). UNITE QIIME release for Fungi [Data set]. UNITE Commun.
Amaresan, N., Kumar, K., Naik, J. H., Bapatla, K. G., and Mishra, R. K. (2018). “Streptomyces in plant growth promotion: mechanisms and role,” in New and future developments in microbial biotechnology and bioengineering, eds B. P. Singh, V. K. Gupta, and A. K. B. T.-N. Passari (Amsterdam: Elsevier), 125–135.
Bengtsson-Palme, J., Ryberg, M., Hartmann, M., Branco, S., Wang, Z., Godhe, A., et al. (2013). Improved software detection and extraction of ITS1 and ITS 2 from ribosomal ITS sequences of fungi and other eukaryotes for analysis of environmental sequencing data. Methods Ecol. Evol. 4, 914–919. doi: 10.1111/2041-210X.12073
Bhattacharyya, D., and Lee, Y. H. (2016). The bacterial community in the rhizosphere of Kimchi cabbage restructured by volatile compounds emitted from rhizobacterium Proteus vulgaris JBLS202. Appl. Soil Ecol. 105, 48–56. doi: 10.1016/j.apsoil.2016.03.020
Bhattacharyya, D., Duta, S., Yu, S. M., Jeong, S. C., and Lee, Y. H. (2018). Taxonomic and functional changes of bacterial communities in the rhizosphere of kimchi cabbage after seed bacterization with Proteus vulgaris JBLS202. Plant Pathol. J. 34:286. doi: 10.5423/PPJ.OA.03.2018.0047
Chen, C., Wang, M., Zhu, J., Tang, Y., Zhang, H., Zhao, Q., et al. (2022). Long-term effect of epigenetic modification in plant–microbe interactions: modification of DNA methylation induced by plant growth-promoting bacteria mediates promotion process. Microbiome 10, 1–19. doi: 10.1186/s40168-022-01236-9
de Salamone, I. E., Di Salvo, L. P., Escobar Ortega, J. S., Boa Sorte, P. M., Urquiaga, S., and Teixeira, K. R. (2010). Field response of rice paddy crop to Azospirillum inoculation: physiology of rhizosphere bacterial communities and the genetic diversity of endophytic bacteria in different parts of the plants. Plant Soil 336, 351–362. doi: 10.1007/s11104-010-0487-y
Di Salvo, L. P., Cellucci, G. C., Carlino, M. E., and de Salamone, I. E. G. (2018a). Plant growth-promoting rhizobacteria inoculation and nitrogen fertilization increase maize (Zea mays L.) grain yield and modified rhizosphere microbial communities. Appl. Soil Ecol. 126, 113–120. doi: 10.1016/j.apsoil.2018.02.010
Di Salvo, L. P., Ferrando, L., Fernández-Scavino, A., and García de Salamone, I. E. (2018b). Microorganisms reveal what plants do not: wheat growth and rhizosphere microbial communities after Azospirillum brasilense inoculation and nitrogen fertilization under field conditions. Plant Soil 424, 405–417. doi: 10.1007/s11104-017-3548-7
Fazal, A., Yang, M., Wen, Z., Ali, F., Ren, R., Hao, C., et al. (2021). Differential microbial assemblages associated with shikonin-producing Borage species in two distinct soil types. Sci. Rep. 11:10788. doi: 10.1038/s41598-021-90251-1
Gao, L., Ma, Y., Zhao, W., Wei, Z., Gleason, M. L., Chen, H., et al. (2015). Three new species of Cyphellophora (Chaetothyriales) associated with sooty blotch and flyspeck. PLoS One 10:e0136857. doi: 10.1371/journal.pone.0136857
Ge, Z., Du, H., Gao, Y., and Qiu, W. (2018). Analysis on metabolic functions of stored rice microbial communities by BIOLOG ECO microplates. Front. Microbiol. 9:1375. doi: 10.3389/fmicb.2018.01375
Harsonowati, W., Marian, M., and Narisawa, K. (2020). The effectiveness of a dark septate endophytic fungus, Cladophialophora chaetospira SK51, to mitigate strawberry Fusarium wilt disease and with growth promotion activities. Front. Microbiol. 11:585. doi: 10.3389/fmicb.2020.00585
Hou, L. W., Groenewald, J. Z., Pfenning, L. H., Yarden, O., Crous, P. W., and Cai, L. (2020). The phoma-like dilemma. Stud. Mycol. 96, 309–396. doi: 10.1016/j.simyco.2020.05.001
Jiménez, J. A., Novinscak, A., and Filion, M. (2020). Inoculation with the plant-growth-promoting rhizobacterium Pseudomonas fluorescens LBUM677 impacts the rhizosphere microbiome of three oilseed crops. Front. Microbiol. 11:569366. doi: 10.3389/fmicb.2020.569366
Ke, X., Feng, S., Wang, J., Lu, W., Zhang, W., Chen, M., et al. (2019). Effect of inoculation with nitrogen-fixing bacterium Pseudomonas stutzeri A1501 on maize plant growth and the microbiome indigenous to the rhizosphere. Syst. Appl. Microbiol. 42, 248–260. doi: 10.1016/j.syapm.2018.10.010
Khan, A. L., Hamayun, M., Ahmad, N., Waqas, M., Kang, S. M., Kim, Y. H., et al. (2011). Exophiala sp. LHL08 reprograms Cucumis sativus to higher growth under abiotic stresses. Physiol. Plant. 143, 329–343. doi: 10.1111/j.1399-3054.2011.01508.x
Klindworth, A., Pruesse, E., Schweer, T., Peplies, J., Quast, C., Horn, M., et al. (2013). Evaluation of general 16S ribosomal RNA gene PCR primers for classical and next-generation sequencing-based diversity studies. Nucleic Acids Res. 41, e1–e11. doi: 10.1093/nar/gks808
Koner, S., Chen, J. S., Hsu, B. M., Tan, C. W., Fan, C. W., Chen, T. H., et al. (2021). Assessment of carbon substrate catabolism pattern and functional metabolic pathway for microbiota of limestone caves. Microorganisms 9:1789. doi: 10.3390/microorganisms9081789
Kong, Z., and Liu, H. (2022). Modification of rhizosphere microbial communities: a possible mechanism of plant growth promoting rhizobacteria enhancing plant growth and fitness. Front. Plant Sci. 13:920813. doi: 10.3389/fpls.2022.920813
Kozich, J. J., Westcott, S. L., Baxter, N. T., Highlander, S. K., and Schloss, P. D. (2013). Development of a dual-index sequencing strategy and curation pipeline for analyzing amplicon sequence data on the MiSeq Illumina sequencing platform. Appl. Environ. Microbiol. 79, 5112–5120. doi: 10.1128/AEM.01043-13
Kunin, V., Engelbrektson, A., Ochman, H., and Hugenholtz, P. (2010). Wrinkles in the rare biosphere: pyrosequencing errors can lead to artificial inflation of diversity estimates. Environ. Microbiol. 12, 118–123. doi: 10.1111/j.1462-2920.2009.02051.x
Le, K. D., Kim, J., Nguyen, H. T., Yu, N. H., Park, A. R., Lee, C. W., et al. (2021). Streptomyces sp. JCK-6131 protects plants against bacterial and fungal diseases via two mechanisms. Front. Plant Sci. 12:726266. doi: 10.3389/fpls.2021.726266
Li, W., Jiang, L., Zhang, Y., Teng, D., Wang, H., Wang, J., et al. (2021). Structure and driving factors of the soil microbial community associated with Alhagi sparsifolia in an arid desert. PLoS One 16:e0254065. doi: 10.1371/journal.pone.0254065
Liu, D., Liu, Y., Fang, S., and Tian, Y. (2015). Tree species composition influenced microbial diversity and nitrogen availability in rhizosphere soil. Plant Soil Environ. 61, 438–443. doi: 10.17221/94/2015-PSE
Lombard, L., Van der Merwe, N. A., Groenewald, J. Z., and Crous, P. W. (2015). Generic concepts in Nectriaceae. Stud. Mycol. 80, 189–245. doi: 10.1016/j.simyco.2014.12.002
Naiman, A. D., Latrónico, A., and de Salamone, I. E. G. (2009). Inoculation of wheat with Azospirillum brasilense and Pseudomonas fluorescens: impact on the production and culturable rhizosphere microflora. Eur. J. Soil Biol. 45, 44–51. doi: 10.1016/j.ejsobi.2008.11.001
Nilsson, R. H., Veldre, V., Hartmann, M., Unterseher, M., Amend, A., Bergsten, J., et al. (2010). An open source software package for automated extraction of ITS1 and ITS2 from fungal ITS sequences for use in high-throughput community assays and molecular ecology. Fungal Ecol. 3, 284–287. doi: 10.1016/j.funeco.2010.05.002
Ozimek, E., and Hanaka, A. (2020). Mortierella species as the plant growth-promoting fungi present in the agricultural soils. Agriculture 11:7. doi: 10.3390/agriculture11010007
Piromyou, P., Buranabanyat, B., Tantasawat, P., Tittabutr, P., Boonkerd, N., and Teaumroong, N. (2011). Effect of plant growth promoting rhizobacteria (PGPR) inoculation on microbial community structure in rhizosphere of forage corn cultivated in Thailand. Eur. J. Soil Biol. 47, 44–54. doi: 10.1016/j.ejsobi.2010.11.004
Quast, C., Pruesse, E., Yilmaz, P., Gerken, J., Schweer, T., Yarza, P., et al. (2013). The SILVA ribosomal RNA gene database project: improved data processing and web-based tools. Nucleic Acids Res. 41, D590–D596. doi: 10.1093/nar/gks1219
Rudolph, N., Labuschagne, N., and Aveling, T. A. S. (2015). The effect of plant growth promoting rhizobacteria on seed germination and seedling growth of maize. Seed Sci. Technol. 43, 507–518. doi: 10.15258/sst.2015.43.3.04
Schloss, P. D., Westcott, S. L., Ryabin, T., Hall, J. R., Hartmann, M., Hollister, E. B., et al. (2009). Introducing mothur: open-source, platform-independent, community-supported software for describing and comparing microbial communities. Appl. Environ. Microbiol. 75, 7537–7541. doi: 10.1128/AEM.01541-09
Suárez-Moreno, Z. R., Vinchira-Villarraga, D. M., Vergara-Morales, D. I., Castellanos, L., Ramos, F. A., Guarnaccia, C., et al. (2019). Plant-growth promotion and biocontrol properties of three Streptomyces spp. isolates to control bacterial rice pathogens. Front. Microbiol. 10:290. doi: 10.3389/fmicb.2019.00290
Sugiyama, A., Ueda, Y., Zushi, T., Takase, H., and Yazaki, K. (2014). Changes in the bacterial community of soybean rhizospheres during growth in the field. PLoS One 9:e100709. doi: 10.1371/journal.pone.0100709
Sun, T. R., Cang, L., Wang, Q. Y., Zhou, D. M., Cheng, J. M., and Xu, H. (2010). Roles of abiotic losses, microbes, plant roots, and root exudates on phytoremediation of PAHs in a barren soil. J. Hazard. Mater. 176, 919–925. doi: 10.1016/j.jhazmat.2009.11.124
Touceda-González, M., Brader, G., Antonielli, L., Ravindran, V. B., Waldner, G., Friesl-Hanl, W., et al. (2015). Combined amendment of immobilizers and the plant growth-promoting strain Burkholderia phytofirmans PsJN favours plant growth and reduces heavy metal uptake. Soil Biol. Biochem. 91, 140–150. doi: 10.1016/j.soilbio.2015.08.038
Vergnes, S., Gayrard, D., Veyssière, M., Toulotte, J., Martinez, Y., Dumont, V., et al. (2020). Phyllosphere colonization by a soil Streptomyces sp. promotes plant defense responses against fungal infection. Mol. Plant Microb. Interact. 33, 223–234. doi: 10.1094/MPMI-05-19-0142-R
Wang, R., Zhang, H., Sun, L., Qi, G., Chen, S., and Zhao, X. (2017). Microbial community composition is related to soil biological and chemical properties and bacterial wilt outbreak. Sci. Rep. 7:343. doi: 10.1038/s41598-017-00472-6
Wen, T., Yuan, J., He, X., Lin, Y., Huang, Q., and Shen, Q. (2020). Enrichment of beneficial cucumber rhizosphere microbes mediated by organic acid secretion. Hortic. Res. 7:154. doi: 10.1038/s41438-020-00380-3
White, T. J., Bruns, T., Lee, S. J. W. T., and Taylor, J. (1990). Amplification and direct sequencing of fungal ribosomal RNA genes for phylogenetics. PCR Protoc. Guide Methods Appl. 18, 315–322.
Whitton, M. M., Ren, X., Yu, S. J., Trotter, T., Stanley, D., and Bajagai, Y. S. (2022). Remediation of pasture dieback using plant growth promotant. Agronomy 12:3153. doi: 10.3390/agronomy12123153
Xu, R., Li, T., Shen, M., Yang, Z. L., and Zhao, Z. W. (2020). Evidence for a dark septate endophyte (Exophiala pisciphila, H93) enhancing phosphorus absorption by maize seedlings. Plant Soil 452, 249–266. doi: 10.1007/s11104-020-04538-9
Yu, S. M., and Lee, Y. H. (2013). Plant growth promoting rhizobacterium Proteus vulgaris JBLS202 stimulates the seedling growth of Chinese cabbage through indole emission. Plant Soil 370, 485–495. doi: 10.1007/s11104-013-1652-x
Yuan, J., Zeng, X. Y., Geng, K., Wijayawardene, N. N., Bhat, J. D., Wu, S. P., et al. (2021). Allophoma species (Pleosporales: Didymellaceae) associated with Thunbergia grandiflora in Guangxi Province, China. Biodivers. Data J. 9:e63643. doi: 10.3897/BDJ.9.e63643
Keywords: PGPR, Pseudomonas, rhizosphere diversity, metabolic functions, canola microbiome
Citation: Świątczak J, Kalwasińska A, Szabó A and Swiontek Brzezinska M (2023) Pseudomonas sivasensis 2RO45 inoculation alters the taxonomic structure and functioning of the canola rhizosphere microbial community. Front. Microbiol. 14:1168907. doi: 10.3389/fmicb.2023.1168907
Received: 18 February 2023; Accepted: 17 April 2023;
Published: 05 May 2023.
Edited by:
James T. Tambong, Agriculture and Agri-Food Canada (AAFC), CanadaReviewed by:
Ugo De Corato, Italian National Agency for New Technologies, Energy and Sustainable Economic Development (ENEA), ItalyWalaa K. Mousa, Mansoura University, Egypt
Bartholomew Saanu Adeleke, Olusegun Agagu University of Science and Technology, Nigeria
Copyright © 2023 Świątczak, Kalwasińska, Szabó and Swiontek Brzezinska. This is an open-access article distributed under the terms of the Creative Commons Attribution License (CC BY). The use, distribution or reproduction in other forums is permitted, provided the original author(s) and the copyright owner(s) are credited and that the original publication in this journal is cited, in accordance with accepted academic practice. No use, distribution or reproduction is permitted which does not comply with these terms.
*Correspondence: Joanna Świątczak, joannaswiatczak@doktorant.umk.pl; Agnieszka Kalwasińska, kala@umk.pl